Geoscience Reference
In-Depth Information
increased by employing a multireference station approach. Over the past few years, the use of the
reference station network approach has shown great promise in extending the interreceiver separa-
tion. The implementation of multiple reference stations in a permanent array offers several advan-
tages over the standard single-baseline approach. It improves the accuracy of the mobile (user)
receiver and makes the results less sensitive to the length of the baselines, at the same time acting
as a “filter” for the lower-quality measurements coming occasionally from some stations. Conse-
quently, the precision of the baseline, expressed as its estimated standard deviation, is improved.
The most important contribution of the network solution, as compared to the single baseline case, is
not, however, the improvement in precision, but rather the improved reliability and availability, as
well as the opportunity to model the atmospheric errors (van der Marel, 1998). A commonly used
base station separation in the network ranges between a few kilometers to a few hundreds of kilome-
ters, depending on the applications served, coverage (network extent), and the required accuracy.
The network-based RTK concept can be summarized as follows: a network of permanent track-
ing stations uses GPS data to estimate the ionospheric and tropospheric corrections in real time and
broadcasts this information to the users, who require accuracy better than DGPS. Thus, network-
based positioning (either RTK or postprocessed) is a three-step procedure: (1) network solution
(no user data is involved) to estimate the instantaneous ionospheric corrections and tropospheric
delays (tropospheric total zenith delay, TZD) per station, (2) transmission of the corrections to the
users (Internet, beacon, mobile phone), and (3) user positioning solution (in relative mode) using
data from a single or multiple reference stations together with the atmospheric corrections derived
in step (1). Naturally, if RTK mode is used, the user needs a data transmit from a reference station
to form a double-difference carrier phase observable for positioning solution (Grejner-Brzezinska
et al., 2005a, 2005b; Kashani et al., 2007; Wielgosz et al., 2004, 2005). This approach is similar
to the virtual reference station (VRS) concept, but without the actual geometric relocation of the
observation to the vicinity of the user receiver (van der Marel, 1998; Wanninger, 2002).
The VRS system uses observations from multiple reference stations to estimate the systematic
errors in the data and to create a unique virtual reference station for each user's location. A synthetic
data set (at the VRS location) is sent to the user located in the vicinity of VRS and is then used
for relative positioning solution. The systematic
errors modeled include ionosphere, troposphere,
satellite orbit errors, and multipath; data are
delivered to the rover in RTCM/CMR+ format.
The following infrastructure is required for
Trimble VRS™: dual-frequency GPS receivers,
PCs, software, and communications links that
are permanently or semipermanently stationed
to provide continuous data logging, monitoring
and data broadcast, rover receiver, rover inter-
face, and office software that handles the data.
The following are the basic processing steps (see
Figure 9.14 and Figure 9.15):
VRS
NMEA
Server
fIGURe 9.14
Virtual reference station (VRS) data
flow. (Courtesy Trimble Navigation Ltd.)
•
Reference station data streams back to
server through LAN, Internet, or radio
links
Roving receiver sends an NMEA string back to the server using cellular modem
•
•
VRS position is established for each user location
•
Server uses VRS position to create corrected observables and broadcasts them to the rover
•
Rover surveying in normal RTK mode but data are relative to the VRS
•
User receiver logs files for postprocessing
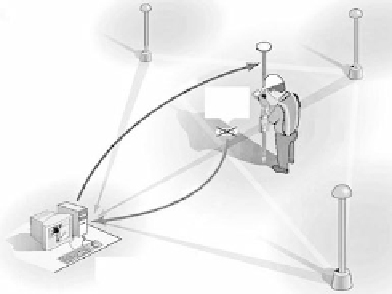