Geoscience Reference
In-Depth Information
0
0
0.9
0.7
0.5
0.3
0.1
-0.1
-0.3
-0.5
-0.7
-0.9
0.9
0.7
0.5
0.3
0.1
-0.1
-0.3
-0.5
-0.7
-0.9
250
250
500
500
750
750
1000
1000
1250
1250
1500
1500
0
116
232
x
348
464
0
116
232
348
464
/
L
D
x
/
L
D
10
−5
m
2
/
s) for the simulation of a stably
stratified flow with uniform bottom heating and boundary layer friction at the bottom and the lateral walls of an equatorial
beta plane. Left: Simulation result with a flux form Eulerian scheme, where the lateral boundary oscillation has been stopped
at nondimensional time
t
∗
= 762 and the model restarted. Right: Simulation result when the model is continued using a semi-
Lagrangian scheme. Note that the data displayed in both panels are identical up to
t
∗
= 762. The Hovmöller data have been
averaged over the near-equatorial region
Figure 9.6.
Hovmöller diagrams of the temporal anomaly of velocity potential (
×
0.844
L
D
from the mid-channel and lowpass filtered to attenuate all frequencies larger
and equal to the beat frequency of the boundary oscillation. Both time and the zonal length are nondimensionalized using the
internal Rossby radius of deformation
L
D
.
±
Available Potential Energy (APE) model intercomparison
[
Blackburn et al.
, 2013;
Williamson et al.
, 2011] for tempo-
ral anomalies of precipitation.
In summary, the presented examples serve as a com-
pelling reminder of the multiscale nature of atmospheres
and oceans, the decisive role of small-scale fluctuations,
and the numerical intricacies associated with the simu-
lation of spatial or temporal anomalies. Certainly many
questions remain, for example, regarding the interaction
of convection and large-scale atmospheric waves and the
role of atmospheric turbulence in general. But it has been
shown that (I)LES and DNS of laboratory analogues can
complement a hierarchy of reduced models, to narrow
the gap between the theoretical understanding of Earth's
climate system, and the growing complexity of com-
prehensive global-scale weather and climate simulations
[
Held
, 2005].
Monogr. Ser.,
vol. 190, editors by L. M. Polvani, A. H. Sobel
D. W. Waugh, pp. 109-121, AGU, Washington, D. C.
Andrews, D. G., J. R. Holton, and C. B. Leovy (1987),
Middle
AtmosphereDynamics
,
InternationalGeophysicsseries
, vol. 40,
Academic, London.
Baldwin, M. P., et al. (2001), The quasi-biennial oscillation,
Rev.
Geophys.
,
39
(2), 179-229.
Blackburn, M., et al. (2013), The aqua planet experiment (APE):
Control SST simulation,
J. Met. Soc. Japan
, special Issue,
The Aqua Planet Experiment (APE),
J. Met. Soc. Japan, 91A
,
pp. 17-56.
Booker, J. R., and F. P. Bretherton (1967), The critical layer
for internal gravity waves in a shear flow,
J. Fluid Mech.
,
27
,
513-539.
Bretherton, F. P. (1966), The propagation of groups of inter-
nalgravitywavesinashearlow,
Q. J. R. Meteorol. Soc.
,
92
,
466-480.
Charbonnel, C., and S. Talon (2005), Influence of gravity waves
on the internal rotation and Li abundance of solar-type stars,
Science
,
309
, 2189-2191.
Charney, J. (1963), A note on large-scale motions in the tropics,
J. Atmos. Sci.
,
20
, 607-609.
Domaradzki, J., Z. Xiao, and P. Smolarkiewicz (2003), Effective
eddy viscosities in implicit large eddy simulations of turbulent
flows,
Phys. Fluids
,
15
, 3890-3893.
Dunkerton, T. J. (1981), Wave transience in a compressible
atmosphere. Part II: Transient equatorial waves in the quasi-
biennial oscillation.,
J. Atmos. Sci.
,
38
, 298-307.
Dunkerton, T. J. (1997), The role of gravity waves in the
quasi-biennial oscillation,
J.Geophys.Res.
,
102
(D22), 26,053-
26,076.
Acknowledgments.
I am extremely grateful to Dr. Piotr
Smolarkiewicz for his supervision, collaboration, enthu-
siasm, and advice on many aspects of the simulations
described in the text as well as for the continued devel-
opment of the EULAG model.
REFERENCES
Alexander, M. J. (2010), Gravity waves in the stratosphere, in
The
Stratosphere: Dynamics, Transport, and Chemistry
,
Geophys.
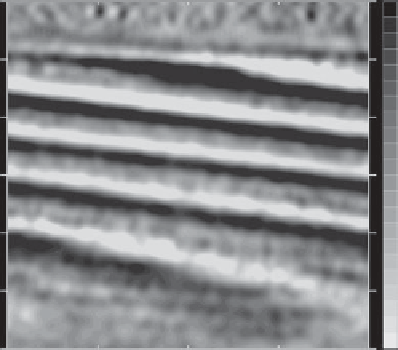















