Geoscience Reference
In-Depth Information
lows are found south of India, near Antarctica
(south of New Zealand) and south of Australia.
The locations of the mass anomalies responsible
for these lows are probably in the lower man-
tle. Many shield areas are in or near geoid lows,
some of which are the result of deglaciation and
incomplete rebound. The thick continental crust
would, by itself, raise the center of gravity of con-
tinents relative to oceans and cause slight geoid
highs. The thick lithosphere (
PACIFIC PLATE
Geoid
High
BAND OF GEOID LOWS
Geoid
High
PLATE
AFRICAN
150 km) under
continental shields is cold, but the seismic veloc-
ities and xenoliths from kimberlite pipes suggest
that it is olivine-rich and garnet-poor; the temper-
ature and petrology have compensating effects
on density. The longterm stability of shields indi-
cates that, on average, the crust plus its under-
lying lithosphere is buoyant. Midocean ridges
show mild intermediate-wavelength geoid highs,
but they occur on the edges of long-wavelength
highs. Hotspots, too, are associated with geoid
highs. The long-wavelength features of the geoid
are probably due to density variations in the
lower mantle and the resulting deformations of
the core--mantle boundary and other boundaries
in the mantle (Richards and Hager, 1984).
Geoid anomalies are expressed as the differ-
ence in elevation between the measured geoid
and some reference shape. The reference shape is
usually either a spheroid with the observed flat-
tening or the theoretical hydrostatic flattening
associated with the Earth's rotation, the equilib-
rium form of a rotating Earth. The latter, used
in Figure 6.2, is the appropriate geoid for geo-
physical purposes and is known as the nonhy-
drostatic geoid. The geometric flattening of the
Earth is 1/298.26. The hydrostatic flattening is
1/299.64.
The maximum geoid anomalies are of the
order of 100 m. This can be compared with the
21 km difference between the equatorial and
polar radii. To a good approximation the net
mass of all columns of the crust and mantle
are equal when averaged over dimensions of a
few hundred kilometers. This is one definition
of isostasy. Smaller-scale anomalies can be sup-
ported by the strength of the crust and litho-
sphere. The geoid anomaly is nonzero in such
cases and depends on the distribution of mass.
A negative
∼
Fig. 6.1
Geoid lows are concentrated in a narrow polar
band passing through Antarctica, the Canadian Shield and
Siberia. Most of the continents and smaller tectonic plates are
in this band. Long-wavelength geoid highs and the larger
plates (Africa, Pacific) are antipodal and are centered on the
equator. The geoid highs control the location of the axis of
rotation. Large-scale mass anomalies in the deep mantle
control the long-wavelength geoid. These in turn can affect
the stress in the surface plates.
order, near-surface mass anomalies that are com-
pensated at shallow depth have no effect on the
geoid.
The shape of the geoid is now known fairly
well, particularly in oceanic regions, because
of the contributions from satellite geodesy [see
geoid images
]. Apart from the geoid highs
associated with subduction zones, there is little
correlation of the long-wavelength geoid with
such features as continents and midocean ridges.
The geoid reflects temperature and density vari-
ations in the interior, but these are not simply
related
to
the
surface
expressions
of
plate
tectonics.
The largest departures of the geoid from
a radially symmetric rotating spheroid are the
equatorial and antipodal geoid highs centered on
the central Pacific and Africa (Figures 6.1 and 6.2).
The complementary pattern of geoid lows lie in a
polar band that contains most of the large shield
regions of the world. The largest geoid highs of
intermediate scale are associated with subduc-
tion zones. The most notable geoid high is cen-
tered on the subduction zones of the southwest
Pacific near New Guinea, again near the equa-
tor. The equatorial location of geoid highs is not
accidental; mass anomalies in the mantle control
the moments of inertia of the Earth and, there-
fore, the location of the spin axis and the equa-
tor. The largest intermediate-wavelength geoid
ρ
, caused for example by thermal
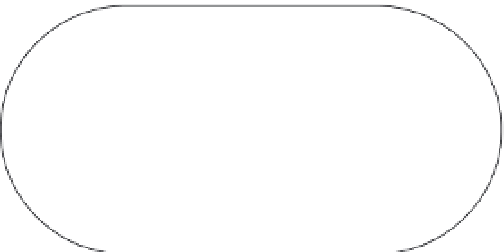
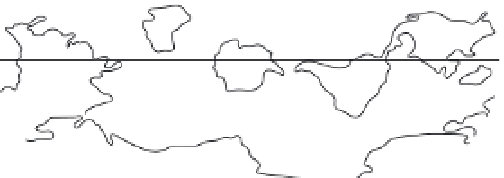







