Geoscience Reference
In-Depth Information
instead begin to determine the response in the diversity of the phytoplankton com-
munity and the quality of the food that they provide.
An alternative model approach is to simplify much of the biochemistry back to a
complexity that we can support with data, and instead focus the computational
power on simulating several tens or hundreds of species (Follows et al.,
2007
), with
parameter ranges set by what we know is biogeochemically possible, providing a
continuum of phytoplankton through the functional types. The resulting ecosystem
structure then emerges out of competition between the species within the physical
and chemical environment, in a way which is more flexible in its ability to respond to
changes in the physical forcing. Combining this approach with more fundamental
descriptions of cellular processes is a potential direction in modelling phytoplankton
ecosystems. At the same time, the role of a diverse grazer population in providing
top-down constraints on the structure of the phytoplankton community, as described
in
Chapter 5
, would add further strength to our attempts at simulating physical
forcing of the ecosystem. Linking the emergent phytoplankton approach (Follows
et al.,
2007
) with a size-based view of grazing (e.g. Baird,
2010
; Banas,
2011
) has
considerable potential.
11.1.3
Organism size and the scales of fluid motion
Can you imagine life as a 10
m
m cell living in a turbulent fluid, or a 2 mm copepod
trying to catch that 10
m organism? Recall from
Chapter 5
that molecular viscosity
plays an enormous role in determining nutrient supply to the cell and, if motile, its
ability to move through the fluid and interact with food particles. This is currently an
area of fruitful research (e.g. Karp-Boss et al.,
1996
; Visser and Kiørboe,
2006
;
Langlois et al.,
2009
), providing some fundamental, physical understanding of
growth and grazing in pelagic food webs. In many cases this knowledge is able to
reveal the mechanics that control observed traits, such as motility or foraging
strategies. However, these new results have not yet been applied to inform the most
widely used ecosystem models. In future model development, close attention to the
guiding principles of how different sized particles are constrained by the physics of
the surrounding fluid could lead to more realistic descriptions of how organisms are
allowed to operate within the food web.
If we move from single cells and small zooplankton, through to fish larvae and
larger fish, fluid viscosity loses its controlling influence, but the medium to large scale
(10s cm to several metres) motion of vertical turbulent transport, along with the
structure of mean flows (e.g. estuarine flows, tidal rectification, frontal conver-
gences), then become the major environmental control. We have seen several
examples of how mean circulation plays a vital role in an organism's life cycle, as
for example in the larval transport by the frontal jet around Georges Bank (
Fig. 8.21
)
or density-driven flows in the ROFI outside Delaware Bay which ensure the survival
of fiddler crabs (
Fig. 9.14
). These are relatively large scale, persistent flows. The
challenge is perhaps how to make compatible measurements of smaller scale, more
ephemeral flows (e.g. the mesoscale variability of frontal convergences) and the
distribution and behaviour of organisms within them. To some extent, this is a
m
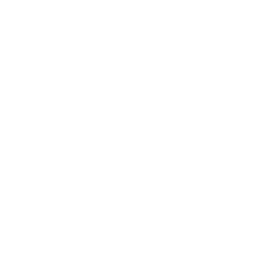

Search WWH ::

Custom Search