Biomedical Engineering Reference
In-Depth Information
Table 11.2 Conventional models predicting the thermal conductivity of solid-
liquid mixtures
Researchers Classical models
Remarks
k
p
k
f
2k
f
þk
p
jðk
p
k
f
Þ
k
eff
Maxwell
(1904)
k
f
¼
1
þ
3j
Depends on the thermal conductivities
of both phases and volume fraction of
solid
k
p
þðn
1
Þk
f
ðn
1
Þ
j
ðk
f
k
p
Þ
k
eff
k
f
¼
Hamilton
and Crosser
(1962)
Valid for both the spherical and
cylindrical particles and n =3/
k
p
þðn
1
Þk
f
þ
j
ðk
f
k
p
Þ
where
y
is the particle sphericity
y
j
k
p
k
eff
k
f
k
eff
2k
eff
þk
f
þ
Bruggeman
(1935)
ð
1
j
Þ
2k
eff
þk
p
¼
0 Valid for spherical particles and
considered interaction between
particles
k
p
þ
2k
f
2j
ð
k
f
k
p
Þ
k
p
þ
k
eff
k
f
¼
Wasp et al.
(1977)
Special case of Hamilton and Crosser's
model with sphericity
2k
f
þ
j
ðk
f
k
p
Þ
=1
y
11.7 Possible mechanisms of k enhancement (after Keblinski et al.
(2002)): (a) enhancement of k due to the formation of highly conductive
layer-liquid structure at liquid/particle interface; (b) ballistic and
diffusive phonon transport in a solid particle; (c) enhancement of k due
to increased effective f of highly conducting clusters.
11.4.1 Effect of liquid layering at the liquid/particle interface
Many researchers have used the concept of a liquid/solid interfacial layer to
explain the anomalous improvement of the thermal conductivity in
nanofluids. Yu and Choi (2003, 2004) suggested models, based on
conventional theory, which consider a liquid molecular layer around the
nanoparticles. The theory proposed the existence of a solid-like nanolayer at
the interface of the solid particle and bulk liquid which acts as a thermal
bridge, thus enhancing thermal conductivity (Fig. 11.8). In these models, the
thermal conductivity and volume fraction of the nanoparticles were replaced
by those of the equivalent particles,
i.e. particles with nanolayers. The
models were formulated as follows.


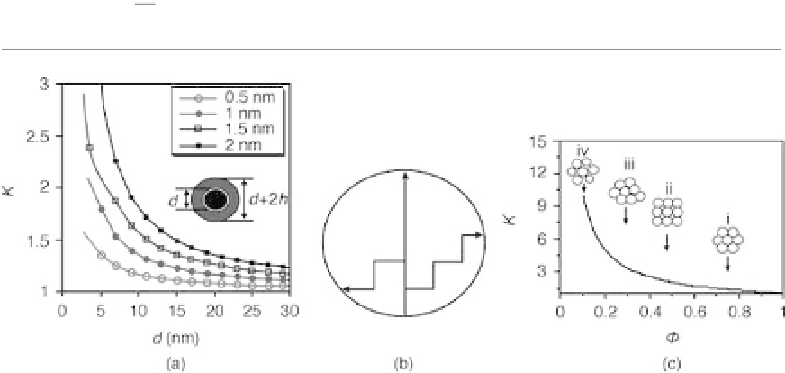




