Biomedical Engineering Reference
In-Depth Information
can be very detrimental to the technique because of the interactions of metals with
charged object in particular through the formation of an electric image that leads to
irreversible sticking [34, 36]. Care has thus to be taken to coat these electrodes to
minimize these effects [34, 36, 37].
10.2.2 The Clausius-Mossoti Factor
If we want to go one step further in the calculation of the force induced by the elec-
tric field, we have to expand the Clausius-Mossoti factor. This discussion is very
different whether we deal with low or high frequencies:
At low frequencies (lower than 10 kHz), there is some dispersion of the dielec-
tric constant of the particle. That is,
e
p
is actually a function of the frequency of the
electric field [38]. This dispersion is mainly due to the relaxation time of the polar-
ization of the double layer surrounding the particle (this time depends on the radius
of the particle and on the Debye length). The situation is quite complex and there is
no satisfactory model that fully describes all the observed phenomena [39].
At higher frequencies, the counterions of the double layer do not have enough
time to move and the particle is basically nondispersive meaning that
e
p
and
s
p
are
independent on the frequency. The polarization is then only due to the contrast in
dielectric constants between the particle and its surrounding medium. This interfa-
cial polarization (Maxwell-Wagner effect [33]) is described by a unique relaxation
time
t
that depends only on the permitivities and conductivities of the particle and
the medium.
We can then express the real part of the Clausius-Mossoti factor:
2 2
ε ε ω τ σ σ
ε ε ω τ σ σ ω τ
(
-
)
(
-
)
p
l
p
l
Re(
f
)
=
+
(10.23)
CM
2 2
2 2
(
+
2 )(1
+
)
(
+
2 )(1
+
)
p
l
p
l
where
ε ε
+
2
2
p
l
τ
=
(10.24)
σ σ
+
p
l
in this case, Re(
f
CM
) varies monotonously with the electric field frequency between
the extreme values
σ σ
σ σ
-
+
ε ε
ε ε
-
+
p
l
p
l
(for
w
®0) and
(
w
®
∞
) (Figure 10.12).
2
2
p
l
p
l
There remain, however, some discrepancies between this expression and the forces
quantitatively measured in diluted colloidal suspensions. It becomes important to
take into account the ionic double layer that effectively modifies the conductivity of
the particles. Some authors have treated this problem by considering an infinitely
thin conductive layer on the surface of the particle. Empirically, one then adds a
surface conductivity l
s
to the particle conductivity
s
p
. The total effective conductiv-
ity then becomes [33]:
σ σ λ
¢
=
+
2
s
a
/
(10.25)
p
p
the behavior of the particles is then well described by using
s
p
¢ rather than
s
p
.

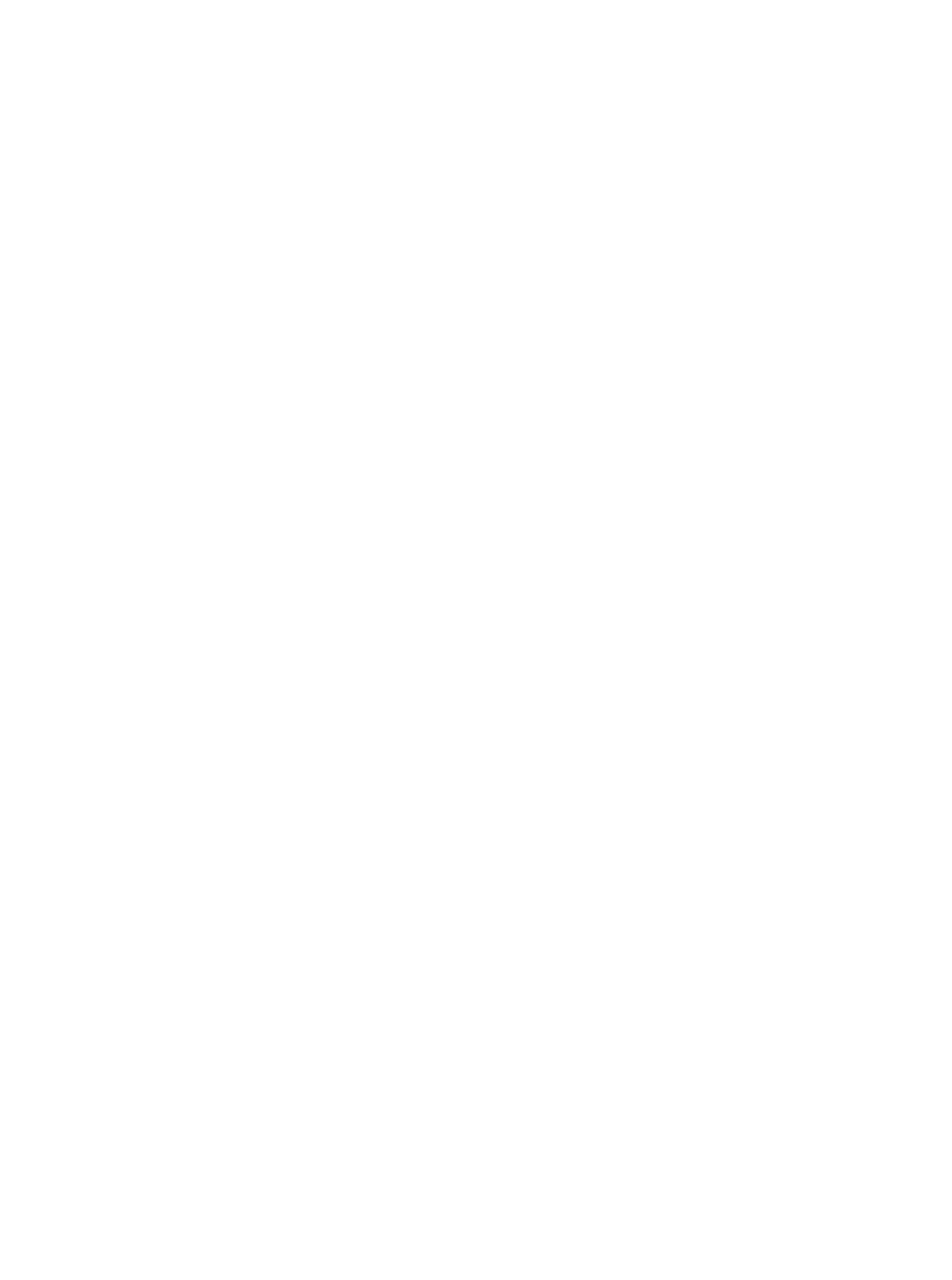


