Agriculture Reference
In-Depth Information
electromagnetic energy from the microwaves into heat and
has several characteristics that differentiate it from con-
ventional heating, such as the following: (1) power can be
turned on and off instantly; (2) it has very rapid dynamics;
(3) it does not rely on contact with hot surfaces or a hot
medium or electrodes; (4) it is selective, that is, different
materials or portions of the same food material having dif-
ferent properties will heat at different rates; and (5) it is
volumetric and thus theoretically more uniform than con-
ventional heating (Coronel et al., 2009).
Microwave (MW) heating of foods is attractive due to
its volumetric origin, rapid increase in temperature, con-
trollable heat deposition, and easy clean-up opportunities
(Ahmed and Ramaswamy, 2008). It is currently being used
for a variety of domestic and industrial food operations and
processing applications. In contrast to conventional heat-
ing, in microwave or dielectric heating, heat is generated
directly inside the material, making possible higher heat
fluxes and thus a much faster temperature rise. However,
heat conduction still plays an important role when heat-
ing thick samples by dielectric heating and for equilibrat-
ing temperatures when heat generation is uneven. Further-
more, microwave heating leads to nonuniform temperatures
inside the food due to different factors depending on mi-
crowave waveguide location, food composition, geometry,
and placement of food inside the oven (Geedipalli et al.,
2007). Design of industrial microwave processes can be
improved with the aid of modeling techniques which re-
late electrical and physical properties of food to microwave
heating performance (Van Remmen et al., 1996).
Microwave heating in foods occurs due to coupling of
electrical energy from an electromagnetic field in a mi-
crowave cavity with the food and its subsequent dissipa-
tion within food product. This results in a sharp increase
in temperature within the product. Microwave energy is
delivered at a molecular level, through the molecular inter-
action with the electromagnetic field, in particular, through
molecular friction resulting from dipole rotation of polar
solvents and from the conductive migration of dissolved
ions (Oliveira and Franca, 2002). The principal mecha-
nisms involved in microwave heating are therefore: dipole
rotation and ionic polarization. Water in the food is the
primary dipolar component responsible for the dielectric
heating. In an AC electric field, the polarity of the field is
varied at the rate of microwave frequency and molecules
attempt to align themselves with the changing field. Heat is
generated rapidly as a result of internal molecular friction.
The second major mechanism of heating with microwaves
is through the polarization of ions as a result of the back
and forth movement of the ionic molecules trying to align
themselves with the oscillating electric field. Microwave
heating is also affected by the state of the constituents,
whether they are bound or free, for example, bound ions
have much lower microwave absorptivities. The volumet-
ric heating rate (
Q
) of microwave at a particular location is
related to the electric field strength by
Q
=
2
π
f
ε
o
ε
E
2
(5.9)
where
f
is the frequency of microwaves,
E
is the strength
of electric field of the wave at that location,
ε
0
is the per-
mittivity of free space (a physical constant), and
ε
is the
dielectric loss factor (a material property called dielectric
property) representing the material's ability to absorb the
wave. In addition to
ε
, there is another dielectric property
parameter called the dielectric constant (
ε
), which affects
the strength of the electric field inside the food.
Application in fruit processing
Juice extraction
Cendres et al. (2011) evaluated the possibility of extract-
ing juice from selected fruits (plum, apricot, grape) us-
ing microwaves and gravity. This process used microwaves
for hydrodiffusion of juice from the inside to the exte-
rior of fruit material and Earth gravity to collect the fruit
juice outside the microwave cavity. Microwave extraction
of fruit juice was performed in a microwave laboratory oven
(2,450 MHz and 1,000 W). A schematic of the experimen-
tal setup is illustrated in Fig. 5.8. A mixture of hot “crude
juice” (in situ water) and steam moved down naturally out-
side the microwave cavity (by an opening on its lower
surface due to gravity and pressure created by steam). The
fruit juice was collected continuously in a graduated cylin-
der or plastic tubes. The extraction was continued until no
more fruit juice was obtained or overheating was detected.
Best yields were obtained at optimized microwave power
and from frozen fruits, respectively. For all three fruits, the
lower the power density tested, the higher the juice yield.
Enzymes
A continuous-flow microwave system was used to pas-
teurize orange juice using PME inactivation and microbial
count as indices (Nikdel et al., 1993). The inactivation of
PME was found to be more pronounced using microwaves
as compared to conventional heating. The kinetics of PME
inactivation in orange juice during microwave heating in
continuous as well as batch mode were compared with
those during conventional heating by Tajchakavit and Ra-
maswamy (1995, 1997), and they found largely enhanced
inactivation of PME while employing microwave heat-
ing. Under both batch and continuous flow microwave
heating conditions, the PME inactivation rate was signif-
icantly higher than in conventional thermal treatment at
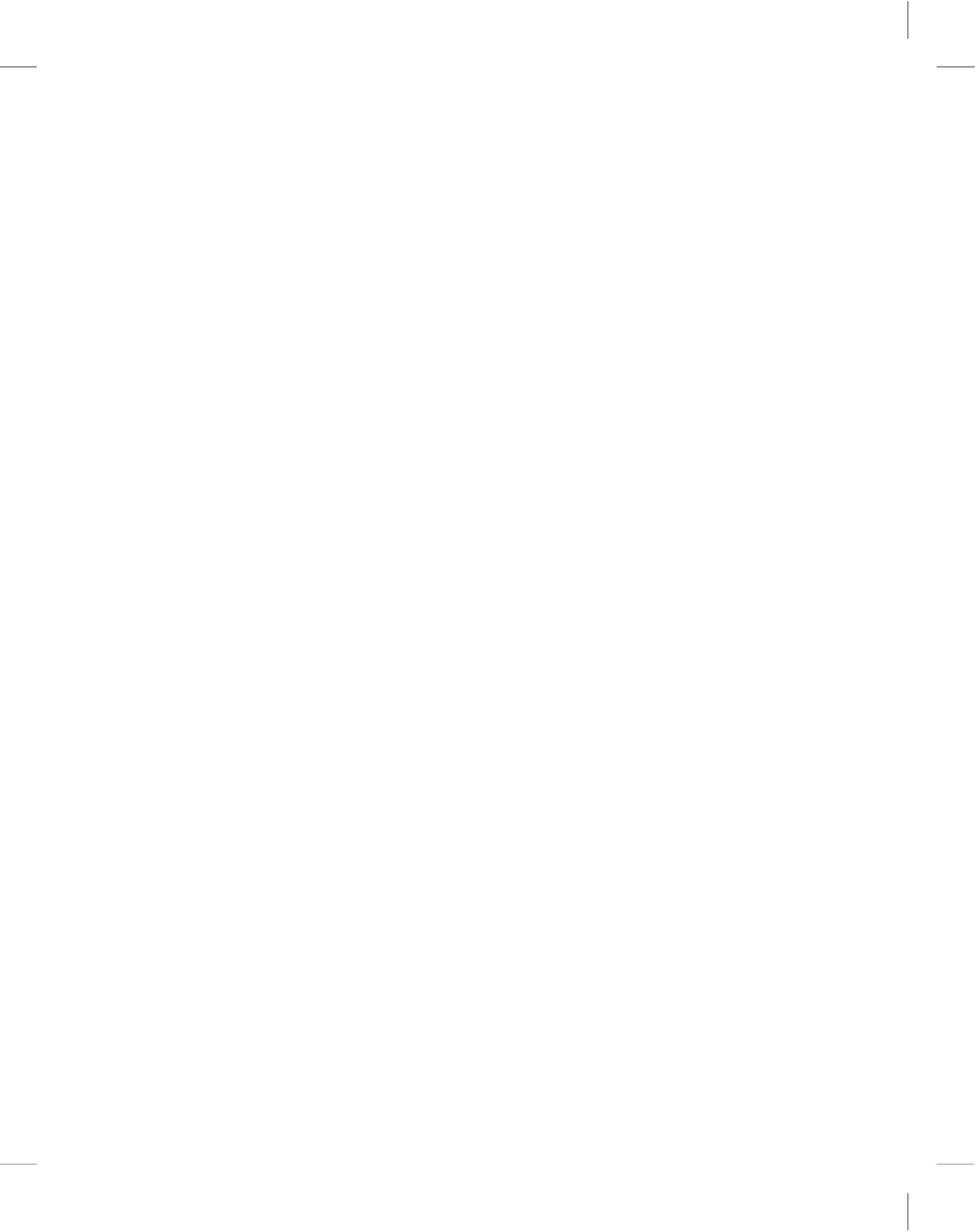
Search WWH ::

Custom Search