Biomedical Engineering Reference
In-Depth Information
or capacitively coupled sensors, a 10-M
resistor to the isolated ground plane from each
input should be used. When ac coupling is desired, IC4A, R8, and C5 are used to o
set
IC1's reference to suppress a baseline composed of components in the range dc to 0.48 Hz.
Also for ac coupling, any remaining baseline at IC1's output may be eliminated by a high-
pass
ff
3 dB) formed by C6 and R9.
IC1's output signal is ampli
fi
filter (1.59 Hz at
fi
ed by IC4b. Notice that the gain of this stage is
fi
xed at
101. Galvanic isolation is provided by IC3, a Burr-Brown ISO107 isolation ampli
fi
er IC.
This type of IC resembles an operational ampli
er but is designed with an internal isola-
tion barrier between its input and output pins. The ISO107's signal channel has a small-
signal bandwidth of 20 kHz and provides an isolation barrier rated at a continuous 2500 V.
In addition to providing a signal channel across the isolation barrier, the ISO107 has an
internal dc/dc converter which powers the isolated side of the ISO107 circuitry and pro-
vides isolated power (
fi
15 mA typical) for the rest of the circuitry of the applied
part (i.e., IC1 and IC2). The isolation rating of the barrier for the dc/dc converter is the
same as that for the signal channel. In total, the 60-Hz leakage current through IC3 does
not exceed 2
15 V at
A with 240 V ac applied across its isolation barrier. The output gain of IC3
is selected through jumpers JP7-JP9 to provide gains of 1, 10, or 100. IC3's output is then
low-pass
fre-
quency of 300 Hz. You may recalculate these values to match the bandwidth required by
your application.
In one position of SW1, the
fi
filtered by IC4C. With the component values shown, the
fi
filter has a cutoff
ff
fi
filter's output is bu
ff
ered directly by IC4D and presented to
the output of the biopotential ampli
fi
er. In the other position, SW1 redirects the output of
IC4C to a tunable-frequency notch
ered by IC4D. This makes it easy
to eliminate 50/60-Hz power line hum that may have been picked up through common-
mode imbalances between the di
fi
filter before being bu
ff
erential patient connections.
As shown up to this point, patient leakage and auxiliary currents have been kept within
allowed limits by virtue of appropriate selection of the components for the circuit. However,
appropriate layout and interconnection are as important in ensuring a safe design. To do so,
every conductive point belonging to the isolated portion of the circuit must be separated from
every conductive point in the nonisolated side of the circuit by the required air clearance and
creepage distances corresponding to reinforced insulation at the rated working voltage. The
layout of a prototype instrument that incorporates this ECG ampli
ff
er is shown in Figure 3.3.
Since there is a 30-mm separation between the closest pins across the ISO107 isolation
barrier, and considering that the internal isolation barrier is rated at a continuous 2500 V
at 60 Hz, the standards would consider this barrier to be equivalent to 1000 V ac-rated rein-
forced insulation. This separation would also be needed between all other isolated and
nonisolated points of the circuit. Most commonly, a biopotential ampli
fi
er is operated in
environments where the power line voltage is the highest potential of concern and has a
maximum rated value of 240 V
RMS
. According to Table 3.1, this would require an air clear-
ance of 5 mm and an 8-mm creepage distance. Remember that these distances also apply
to the separation of any point on the isolated side and any conductive fastening means in
connection with any nonisolated part of a medical instrument.
Amplifying the electrical activity produced by the heart introduces a number of addi-
tional requirements addressed by the front-end protection circuit shown in Figure 3.2.
Physicians conducting electrophysiological diagnosis and therapy of conditions involving
the heart assume the possibility of ventricular
fi
fi
fibrillation during a procedure. Reverting
fi
y
forcing high current through the heart. To overcome tissue resistivity, this implies the
delivery of a high-energy, high-voltage pulse.
Typical external de
fibrillation back into a normal rhythm driven by the sinus node of the heart involves brie
fl
fi
brillators deliver this pulse by discharging a 32-
F capacitor charged
up to 5000 V dc through a 500-
H inductor directly into paddle electrodes placed on the
chest of the patient, who may be assumed to act as a 100-
resistor. A sizable fraction of
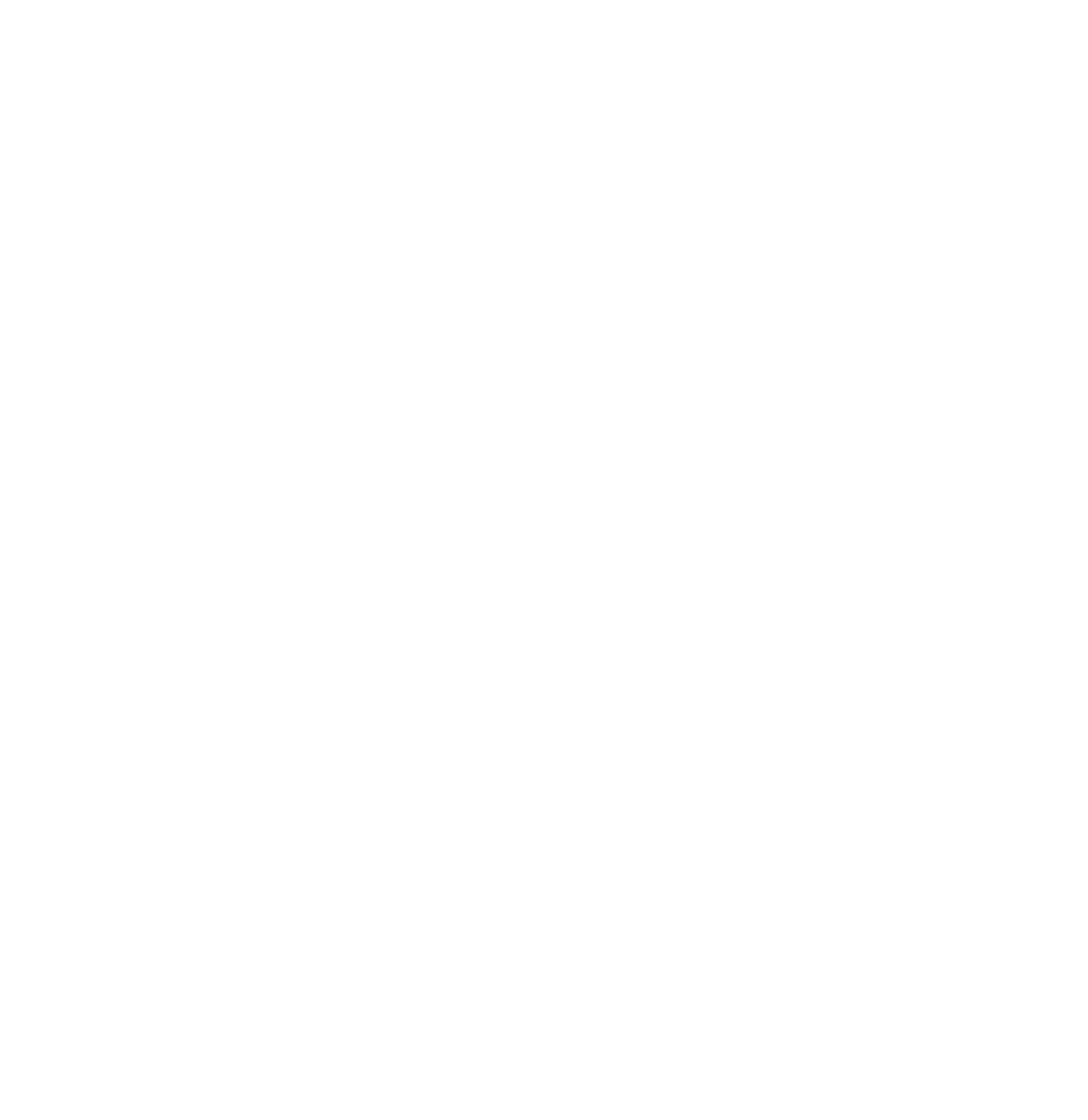
Search WWH ::

Custom Search