Biomedical Engineering Reference
In-Depth Information
which hydrate in an aqueous solution at temperatures below the lower critical
solution temperature (LCST). When hydrated, the polymer chains form extended
structures, while above LCST, which is about 32
ı
C, the polymer chains dehy-
drate and collapse. So, below LCST, microtubules are prevented from binding to
kinesin motors and diffusing in solution while binding, and hence, microtubule
gliding on kinesin is allowed above LCST. The switching of the microtubule
motility is reversible. However, not all microtubules behave in the same way when
the temperature is cyclically changed. At cooling, the shorter microtubules are
predominantly released since polymer chain hydration is accompanied by partial
screening of kinesin molecules so that the effective distance between adjacent
kinesin motors increases. Therefore, continuous microtubule gliding, which implies
contact with at least two kinesin molecules at any given moment, is possible only
for longer microtubules; this device sorts the molecules according to their lengths
as temperature varies.
Although most artificial biomotors rely on the kinesin/microtubule interactions,
there are also nanomotors involving myosin and actin filaments. In particular, silicon
nanowires functionalized with the myosin motor domain and dispersed in aqueous
solution confine the motion of actin filaments along their circumference (
Byun et al.
2009
). These filaments move ballistically on the silicon nanowires over distances as
long as 100m.
Guided unidirectional movement of microtubules gliding on kinesin can be
achieved in microfabricated channels, as those in Fig.
6.13
(
Lin et al. 2006
).
Although initially moving diffusively in solution, all microtubules placed on the
structure acquire a rotational movement in the same direction, the arrowhead or
rounded corners acting as motion rectifiers for the microtubules that follow the
kinesin-coated sidewalls of the channel. When a microtubule encounters a sidewall,
it must locally bend to glide along the sidewall and tends to reach the outer sidewall
of the channel, the bending energy increasing with the sharpness of the channel
edges. For bending energies larger than the binding energy between microtubule and
kinesin, the former can detach. On the contrary, curved ends of the rectifier regions,
such as those in Fig.
6.13
b, c, redirect more efficiently the gliding microtubules
toward the circular channel. The configuration in Fig.
6.13
c is the most effective in
collecting microtubules in the channel and thus sorting them from solution since the
a
b
c
Fig. 6.13
Microfabricated channel geometries that rectify the motion of microtubules: (
a
) arrow-
headed and (
b
,
c
) rounded corner rectifiers
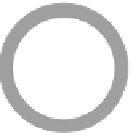




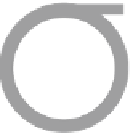







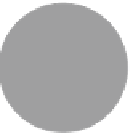













Search WWH ::

Custom Search