Biomedical Engineering Reference
In-Depth Information
Figure 9.5
Application of RDCs in the analysis of RNA dynamics. (A) RDC-derived
local motions of internal loop residue C27 (black) in theophylline-RNA
complex. Reproduced from ref.
147
with permission. # American Chemical
Society, 2001. (B) Global motions observed using RDCs involving
correlated changes in the inter-helical twist (a and c) and bend (b) angles.
Reproduced from ref.
71
with permission. (C) Secondary structure (left) and
NMR structure of human telomerase P2ab (PDB ID 2L3E, right).
Superposition of the order tensor frames yields an average inter-helical
angle of 89u, and internal generalised degree of order q
int
y0.69 (where q
int
ranges from 0-1, with 0 being flexible and 1 being rigid). (D) Conformers
from atomic-resolution dynamics ensemble of HIV-1 TAR (grey)
constructed by combining domain-elongation RDCs and molecular
dynamics simulations reveals very high similarity to those observed in
ligand bound states (orange). Reproduced with permission from ref. 16.
trinucleotide bulge.
127,149
Discrete ensemble analysis of RDCs measured in two
domain elongated TAR RNA constructs [Figure 9.4(B)] made it possible to
visualise the inter-helical trajectory in 3D. Results revealed a specific trajectory
in which the helices bend and twist in a spatially correlated manner
71
[Figure 9.5(B)]. Thus, while the helices undergo large amplitude collective
motions (.90u), they do not move in a spatially random manner. Importantly,
all of the known ligand-bound TAR conformations fall along various positions
of this dynamic trajectory, indicating that ligands most likely capture pre-
existing TAR conformations by 'conformational selection'. It is important to
note that the inter-helical motions observed in TAR using RDCs are not fully
captured by spin relaxation data, most likely because the motions occur at the
nano- to microsecond timescale that are inaccessible to spin relaxation.
127,150
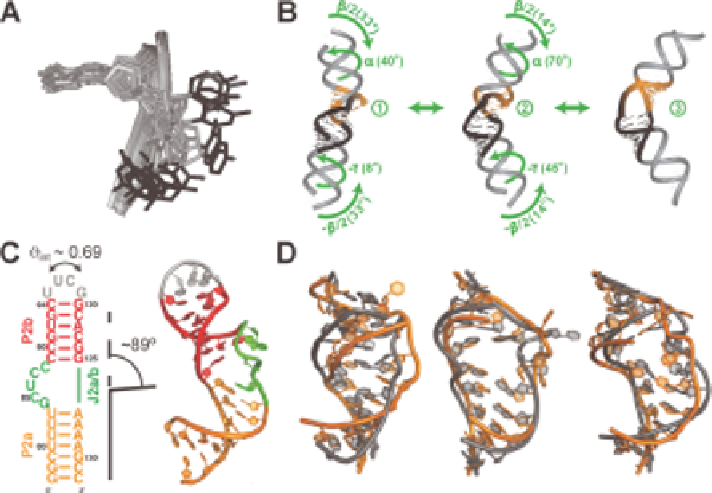