Agriculture Reference
In-Depth Information
Table 5.9
Observed plant heights from both
parents ( P
1
and P
2
), the F
1
, F
2
, generations
and both backcross generations ( B
1
and B
2
)
along with the expected plant height from the
joint scaling test parameters, and the difference
between the observed and expected plant height.
test does more than test the adequacy of the additive-
dominance model. It also provides the '
best
' estimates of
all the parameters required (and their standard errors) to
account for differences among family means when the
model is adequate. If you try to estimate
m
,
]
with the procedure shown earlier you will find values of
m
[
]
[
a
and
d
=
[
]=
[
]=
10.310. These
estimates do not differ markedly from those estimated
(107.322, 8.1997, 10.0587, respectively) from the joint
scaling test. However, the difference may in some cases
be of importance. The joint scaling test can also be
readily extended to more complex situations.
To conclude, in this example the best estimates show
that the additive and dominance components are of
the same order of magnitude and since
107.365,
a
8.925 and
d
Family
Observed
Expected
Obs-Exp
P
1
+
116.300
115.522
0.778
P
2
98.450
99.122
+
0.672
F
1
+
0.294
117.675
117.381
F
2
111.778
112.351
−
0.573
B
1
−
116.000
116.451
0.451
B
2
−
109.161
108.252
0.090
is signifi-
cantly positive, alleles that increase final height must be
dominant more often than alleles that decrease it.
[
d
]
2
have been estimated (i.e.
m
,
[
a
]
and
[
d
]
)
, thus the
χ
value has 6
3 degrees of freedom.
The contribution made to the
−
3
=
2
by P
1
, for exam-
ple, is the difference between Observed and Expected
divided by the variance (or in our case we can multi-
ply by one over the variance that is the weight. So, for
example
χ
What could be wrong with the model
In some instances the results from generation testing will
conclude that an additive-dominant model of inheri-
tance does not adequately account for the data. There
are many possible explanations of this, and here only
three, in order of increasing genetic complexity, will be
mentioned briefly.
2
[
−
]
×
=
0.5862.
Summing the six such contributions, one from each of
the six types of family, gives a
116.300
115.522
0.968
2
of 3.411 for 3 degrees
of freedom, which has a probability of between 0.40 and
0.30. The model must therefore be regarded as adequate
(i.e. there is no evidence of anything beyond additive
and dominance effects).
The individual scaling tests, A, B and C, referred to
earlier can, of course, also be used to test the model.
Thus with the present data
χ
•
Abnormal chromosomal behaviour.
In the case of
single gene
or mu
ltiple gene cases, the predicted
means of the F
1
, B
1
and P
1
generations were derived
in terms of
m
,
[
]
[
]
. Throughout, the assump-
tion was made that a heterozygote contributes equal
proportions of its various gametes to the gene pool
following genetic segregation. If this assumption
is unwarranted. then the frequencies of the differ-
ent genotypes, and therefore their contributions to
the generation means in terms of
m
,
a
and
d
A
=
2B
1
−
P
1
−
F
1
=[
2
×
116.000
]−
116.300
−
117.675
=−
1.975
,
would not be as predicted. This could result from
the elimination of deleterious alleles through gametic
selection.
[
a
]
and
[
d
]
2
2
2
2
F
1
σ
A
=
σ
B
1
+
σ
P
1
+
σ
4
=[
4
×
0.4888
]+
1.0334
+
0.9699
=
3.959
•
Cytoplasmic inheritance.
If a character is deter-
mined, or affected, by non-nuclear genes, then the
character will depend on which of the genotypes
was maternal. Under such circumstances, inheritance
cannot be explained fully in terms of
m
,
σ
A
=
√
(
)
=
leading to
3.959
1.990
=−
±
1.99 which, when compared in
Student
t
statistical tables does not differ significantly
from the value 0 expected.
The joint scaling test gives exactly the same answer as
the A-, B- or C-scaling tests. However, the joint scaling
Thus A
1.98
[
]
[
]
a
and
d
.
•
Non-allelic interactions or epistasis.
We have already
mentioned the phenomenon of genetic dominance.
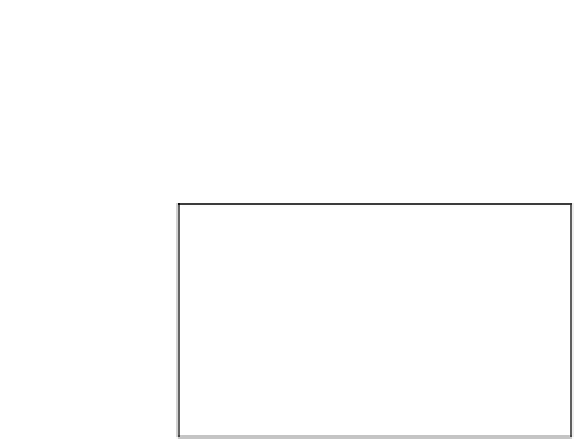









