Biomedical Engineering Reference
In-Depth Information
From the results described earlier it appears that sophisticated software implementa-
tion, such as those based on neural network (NN) algorithm could be utilized for
recovering an analytical signal derived from a multisensing application. Many examples
were proposed for gas sensing but not so many for ion screening in solution. We are opti-
mizing our NN for a better discrimination of metal traces, by changing some parameters
and some strategies and we hope that a software solution will eliminate the need to intro-
duce modifications in the proposed analyzer, such as the use of a modified working elec-
trode (with a mercury layer or with some chemical agents, such as chelant compounds or
resins) or some sample treatments or more sophisticated electronics.
9.3
Liquid Sensing Using Polymer and Nanocomposite Matrices
Novel nanomaterials for use in bioassay applications represent a rapidly advancing field.
Various nanostructures have been investigated to determine their properties and possible
applications in biosensors. These structures include nanotubes, nanofibers, nanorods,
nanoparticles, and thin films. Of these, nanoparticles are the best-studied structures. The
biosensors based on different kinds of nanostructures are discussed in this review. A gas
sensor, fabricated by selective growth of aligned carbon nanotubes (CNTs) by pulsed
plasma on Si3N4/Si substrates patterned by metallic platinum, is presented for inorganic
vapor detection at room temperature. Poly(o-anisidine) (POAS) deposition onto the CNTs
device was shown to impart higher sensitivity to the sensor. Upon exposure to HCl the
variation of the CNTs sensitivity is less than 4%, while the POAS-coated CNTs devices
over a higher sensitivity (i.e., 28%). The extended detection capability to inorganic vapors
is attributed to direct charge transfer with electron-hopping effects on intertube conduc-
tivity through physically adsorbed POAS between CNTs.
The CNTs thin film was grown using a radio frequency pulsed plasma-enhanced chemical
vapor deposition (RF-PECVD) system. Prior to the nanotube growth, a Si
3
N
4
/Si substrate
was patterned with platinum film (60 nm thick) by vacuum deposition through shadow
masks, containing rectangular stripes 30
m wide and a back-deposited thin film platinum
heater commonly used in gas-sensor applications. A thin film (3 nm) of Ni catalyst was
deposited onto the Si
3
N
4
/Si substrates using thermal evaporation. The CNTs film was
obtained by pulsed RF-PECVD deposition on Si
3
N
4
/Si substrates as reported previously (66).
POAS was chemically synthesized by oxidative polymerization of the monomers by
using (NH
4
)
2
S
2
O
8
under controlled conditions. For the synthesis, the monomer, previously
distilled, was dissolved in HCl 1M aqueous solution. The oxidant was thus added slowly
maintaining the temperature at 0-4°C by means of an ice bath. The reaction was contin-
ued for about 12 h. The dark green precipitate emeraldine-salt form recovered from the
reaction vessel was filtered and washed with distilled water. The precipitate was subse-
quently treated with a diluted solution of NH
4
OH for 6 h to obtain the crude materials in
the undoped form (blue in color). The precipitate recovered after filtration was washed
again with water and treated first with methyl alcohol and then with ethyl ether for 24 h
to remove the oligomers constituted by few monomer units. The material obtained from
the last filtration was heated at 80°C in a vacuum system to remove the traces of solvents.
The final product contained the emeraldine base form of POAS that was completely solu-
ble in chloroform.
Figure 9.4 shows two distinct groups of
I
-
V
curves resulting from nanotubes and
polymer POAS-coated nanotubes. Each group consists of results from several sensors. It
can be seen from Valentini et al. (3) that the conductivity (slope) is almost the same for

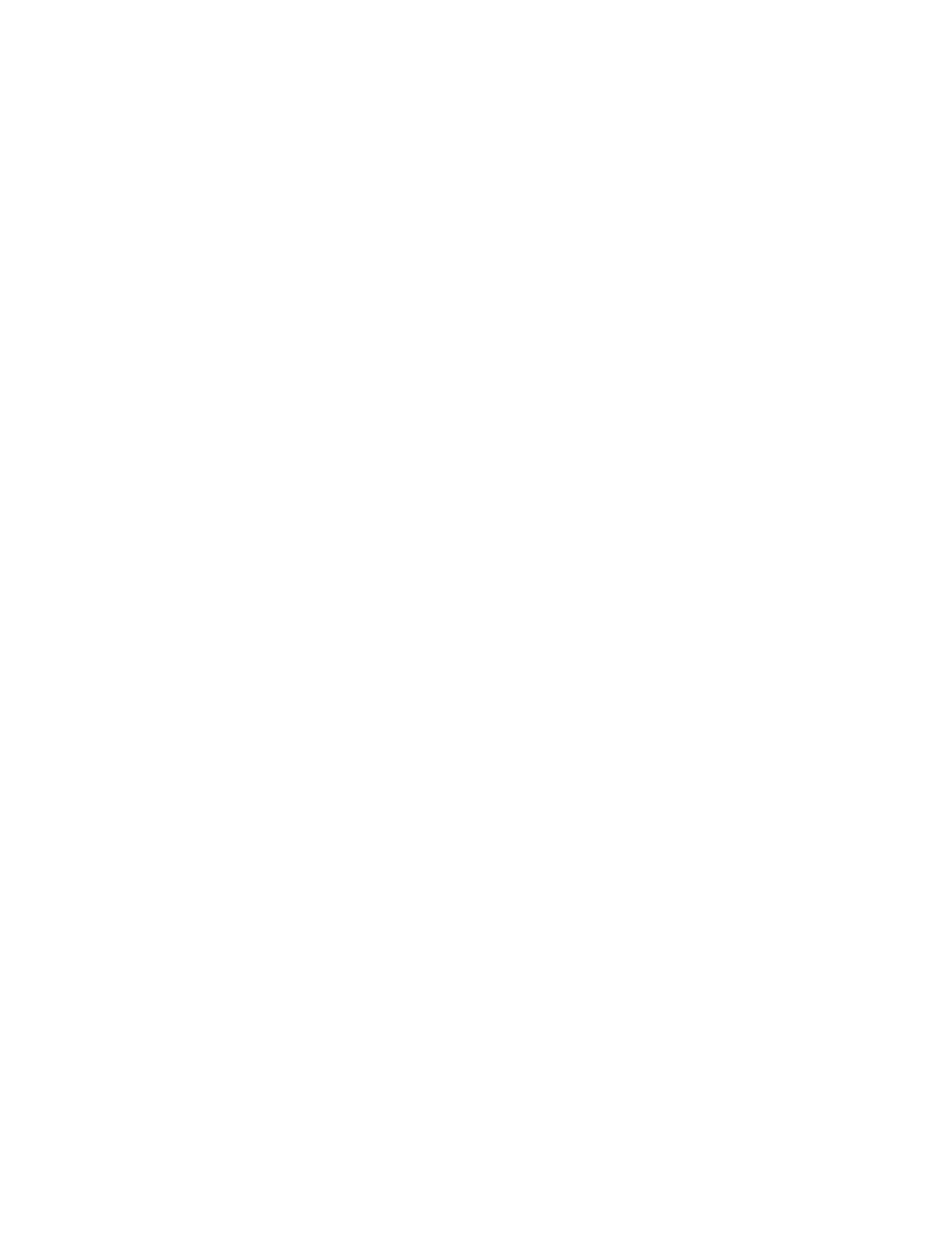