Geology Reference
In-Depth Information
A
C
52
52
47
47
42
42
37
37
32
27
32
27
X
X
′
Y
Y
′
22
-0.15
22
-0.15
-0.1
-0.05 0
Distance (mm)
0.05
0.1
0.15
-0.1
-0.05 0
Disstance (mm)
0.05
0.1
0.15
B
D
X
Y
X
′
Y
′
Figure 14.4.5. A conceptual diagram showing the relationship of the depth of a coal fire to its surface thermal
anomaly. (A) and (B) are cross-sectional and plan views, respectively, of a thermal anomaly from a laboratory
simulation of a shallow fire. (C) and (D) are the cross-sectional and plan views, respectively, of a thermal anomaly
from a laboratory simulation of a relatively deeper coal fire. Shallow buried heat sources generate narrower and
taller thermal anomaly profiles compared to deeper buried heat sources. From Prakash and Berthelote 2007.
Panigrahi et al. (1995) proposed a mathematical relationship between the thermal profile and fire depth, stating that
the full width of the profile at half its maximum intensity (also referred to as Full Width Half Max) approximately
equates to one-third of the fire depth. Berthelote et al. (2008) carried out laboratory simulations of coal fires and
established a more complex empirical relationship between the surface thermal profile and the fire depth, which
marginally outperformed the depth estimates of Panigrahi et al. (1995). For the actual empirical relationship and
comparative performance evaluation of these models, the readers are referred to Prakash and Berthelote (2007).
In real life, both conduction and convection play a role in heat transfer from depth, with convection arguably
playing a more important role. However, modeling the convective component is far more challenging. The
interstitial pore spaces in overlying rock units, cracks, fractures, and faults all constitute convective pathways for
the heat energy to disseminate. Among other factors, the amount of heat energy actually dissipated is a complex
interplay of the intensity of the heat source and the nature, density, and interconnectivity of the convective
pathways. The convective pathways differ spatially and temporally over individual fires, making it difficult to
model heat transfer in the field as well as from remote sensing images.
Prakash et al. (1995b) accounted for the convective component of heat transfer by a very crude assumption that the
convection component of the heat transfer from the subsurface to surface was three to five times greater than heat
transfer by conduction alone. Rosema et al. (1999) modeled the convective component as a single chimney system.
They made the assumption that the integrated effect of all convection pathways was effectively the same as one single
large vent of a certain dimension. Wessling et al. (2008) further analyzed the mechanisms of conduction and convection
pathways that cause thermal anomalies on the surface and numerically modeled the effect of these processes.
Refining these existing models with the intent to estimate depth of the buried-heat source is an area that merits
further research. It is important to note that these models make an effort to mimic natural processes by replacing
them with mathematical representations that are only as accurate as knowledge permits.
Subsidence Mapping
S
ubsidence of the surface, a phenomenon where the ground surface caves in, is not uncommon in coal-mining
areas. If coal is extracted from the subsurface without completely filling the void that the extraction process leaves
behind, or without reinforcing the overlying rock strata in some other manner (e.g., by roof bolts), the mined area
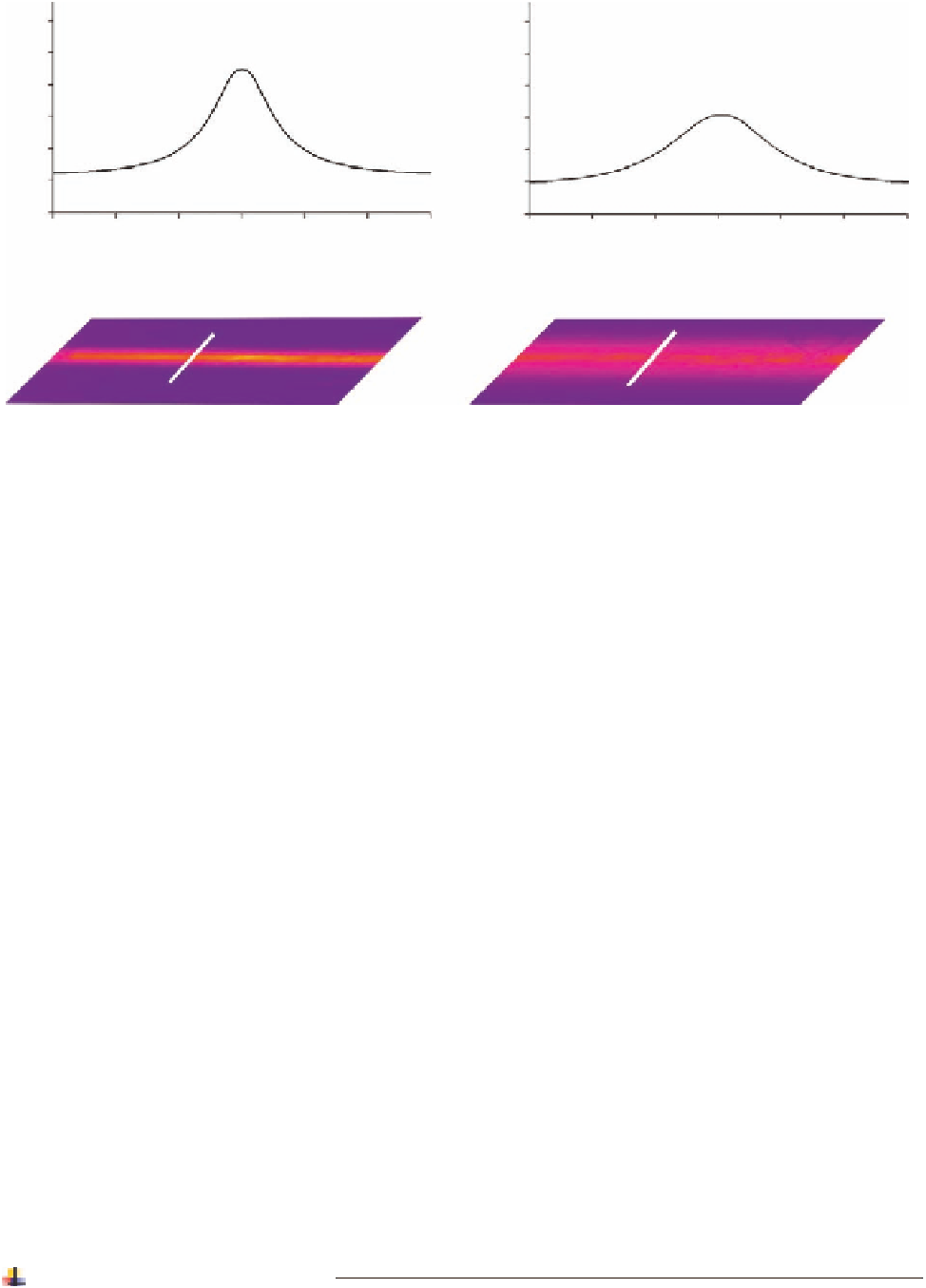