Biomedical Engineering Reference
In-Depth Information
In the final section, we describe a hybrid algorithm for modeling a gen-
eral class of diffusion-reaction systems and its application to mixing-induced
precipitation.
7.2 DPD Models for Biological Applications
When DPD models are used to simulate liquids, the particles overlap exten-
sively (there are tens of particles in the interaction volume of 4
πr
0
/
3, where
r
0
is the cut-off range of the particle-particle interactions). As the number
of atoms,
N
m
,
represented by a DPD particle increases, the thermal energy
(
k
B
T/
2 per degree of freedom, where
k
B
is the Boltzmann constant and
T
is
the temperature), remains constant while the DPD particle-particle interac-
tions increase in magnitude. The decrease in the thermal energy relative to
the interaction energy drives the system through a Kirkwood-Alder transition
(Kirkwood 1939; Alder and Wainwright 1962) from a fluid to a solid, and this
limits the size of the cluster of atoms or molecules that the DPD particles
can represent. For DPD simulations with interaction parameters that have
been selected so that the DPD fluid properties match the properties of real
liquids the DPD particle mass can be no more than 10-100 times the atomic
or molecular mass (Dzwinel and Yuen 2000). Consequently, speedups (rela-
tive to MDs) greater than about 10
5
cannot be achieved using the standard
DPD model. DPD can be regarded as thermostatted nonequilibrium MDs with
soft particle-particle interactions, and the DPD thermostat has been used in
nonequilibrium MDs simulations (Guo et al. 2002). The soft particle-particle
interactions allow much larger time steps to be taken, and this is a more
important advantage than the coarse graining, relative to standard MDs.
Owing to its computational eciency compared to MDs, while a significant
molecular level detail is retained, DPD has been extensively applied to inves-
tigate the effects of the size, shape, and rigidity of large molecules, and their
intermolecular interactions on the behavior of soft condensed matter. This
makes DPD an attractive approach for the simulation of biological systems
on supraatomic length scales and on timescales beyond the MD timescale, but
where the effects of the thermal fluctuations and thermal (entropic) forces are
still important. DPD has been used quite extensively to simulate the dynamic
behavior of cell membranes, and lipid bilayers, which play very important roles
in living cells (Venturoli and Smit 1999; Groot and Rabone 2001; Shillcock and
Lipowsky 2002; Yamamoto et al. 2002; Kranenburg et al. 2004; Ortiz, Nielsen
et al. 2005; Shillcock and Lipowsky 2005; Gao et al. 2007; Revalee et al. 2008;
Wu and Guo 2008). As the computational cost of full MDs simulations of the
self-assembly of membranes is very high, Venturoli and Smit (1999) developed
a DPD model to study the lateral pressure and density variation across bio-
logical membranes. In this coarse-grained DPD model, the hydrophilic head
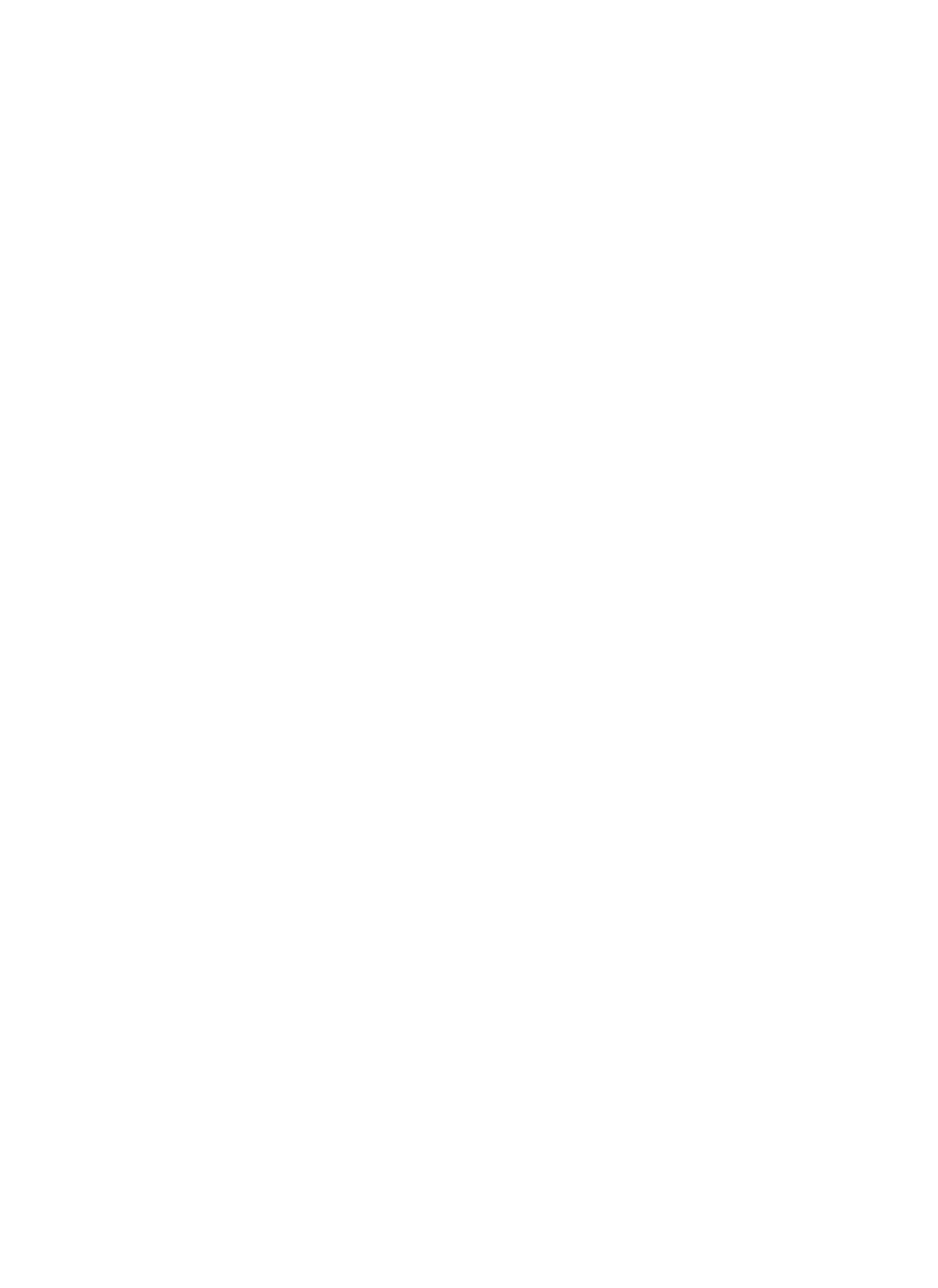
Search WWH ::

Custom Search