Biomedical Engineering Reference
In-Depth Information
metabolism is likely to be influenced by the transport phenomena of nutrient
and waste products that are regulated by the flow, but it may also be directly
affected by the various stresses generated by the fluid motion. Fluid flow within
natural tissues plays important roles in morphogenesis, metabolism function,
and pathogenesis. In the design of new biomaterials mimicking biological tis-
sues, it is well recognized today that three-dimensional
in vitro
culture bet-
ter recapitulates physiological cell environment. Indeed, fluid flow and solid
strain that are imposed within tissue cultured in bioreactors not only affect
cell nourishment but also exert on cells mechanical actions such as pressure
effects, drag interactions, and viscous shear stresses (Chen 2008).
This fact is largely exploited in tissue engineering, a pluridisciplinary
research field based on the employment of biological cell systems to develop
therapeutic strategies aiming at the replacement, repair, maintenance, and
enhancement of tissue function (Lanza et al
.
2002). The general strategy for
tissue engineering then involves seeding cells into a biocompatible porous scaf-
fold and culturing this seeded structure in a bioreactor (Martin et al
.
2004).
Thus, tissue engineering presents sound promise for the next future, provid-
ing a large number of transplantable organoids. Indeed, it requires proposing
challenging development in effective bioreactors to overcome nutrient trans-
port limitations and to subject cells to optimal mechanical stresses.
Current strategies generally take advantage of the porous media morphol-
ogy of the scaffolds generally displaying a high porosity
φ
0
.
5 (volume of
pores divided by the total volume) and large pore length scales (
a
≥
m),
that is to say an order of magnitude larger than the cell length scale. The
use of perfusion bioreactors to force culture fluid to flow through the medium
allows enhancement of nutrient transport and generation of mechanical
stim-
uli
upon the cells (Abousleiman and Sikavitsas 2006; Cimetta et al
.
2007; Kim
et al
.
2007). For small tissue-engineered construct these methods have been
shown to be successful in comparison to “static” culture (Glowacki et al
.
1998;
Goldstein et al
.
2001; Bancroft et al
.
2002; Cartmell et al
.
2003). However,
problems arise when the tissue size is scaled up. Cells residing away from the
inlets and outlets may sit in almost stagnant regions, where both nutrient
delivery and shear stress are compromised. If the center of the construct is
to receive adequate flow, then regions near the inlet and outlet may suffer by
receiving too much shear stress. The nonuniformity of the flow and shear-stress
distributions is problematic (see Figure 3.1).
It avers then very critical to elaborate a comprehensive analysis of the fluid
mechanics and related transport phenomena inside such porous media and the
way they control the different life processes. As presented here, within such
multiscale natural or artificial materials, the dynamic interactions between
the fluid phase and the solid structure as well as the diffusive, convective
transport of nutrient by fluid and oxygen consumption by immersed cells
are phenomena not always completely understood and controlled. Some perti-
nent predictions about the microfluid dynamic environment imposed on three-
dimensional engineered cell systems within bioreactors is expected from such
an analysis.
≈
100
µ
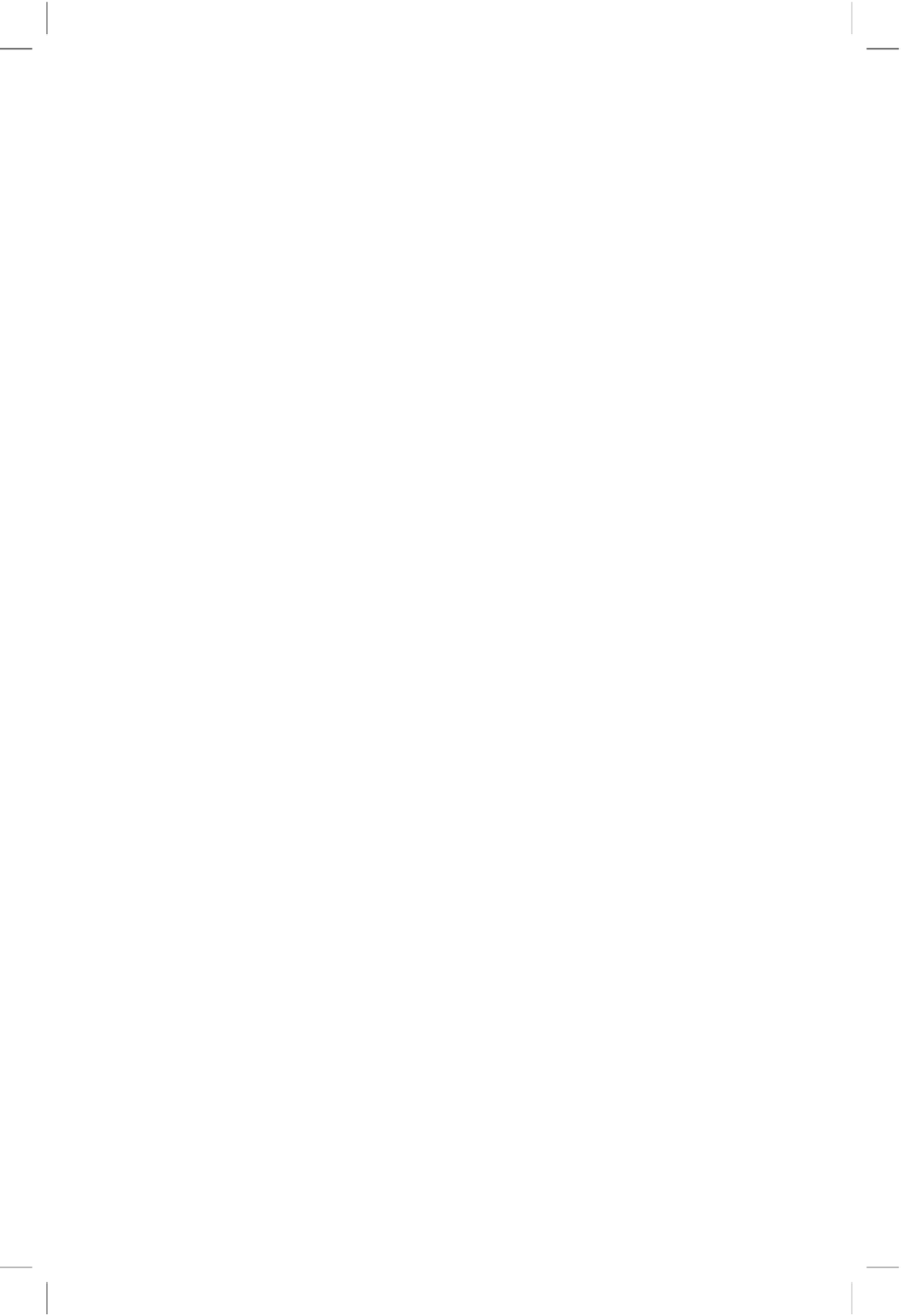
Search WWH ::

Custom Search