Biomedical Engineering Reference
In-Depth Information
FIgurE 9.11
Mouse tail dermis and epidermis. (a) Bright-field image. (b) SHG amplitude (normalized) and (c)
phase (wrapped) reconstructed from a single hologram. All images present the same region of the specimen and
scale bars are 10 μm. (Reprinted from Shaffer, E. et al., 2010b. Label-free second harmonic phase imaging of biologi-
cal specimen by digital holographic microscopy,
Optics Letters
, 35, 4102-4104. With permission of Optical Society
of America.)
image data. This year, Xu et al
.
(2010a) suggested that single molecule SHG would be too weak to account
for the detected SHG signal and that coherent scattering had to play an important role. Observation of
the phase of the SHG signal generated by collagen fibers, originally published in Shaffer et al
.
(2010b)
and reprinted here (Figure 9.11), tends to support this theory.
In this experiment, 5 μm-thick coronal sections of mouse tail were fixed with paraformaldehyde
(PAF) 4%, embedded in paraffin, and observed with both bright-field (Figure 9.11a) and holographic
SHG microscopy, leading to reconstruction of amplitude and phase-contrast images (respectively
Figures 9.11b and 9.11c). All three images were recorded with the same microscope objective and imag-
ing wavelength of 400 nm, using a light-emitting-device for the bright-field image and a 800 nm fun-
damental-wavelength femtosecond laser for the SHG holographic images. Under these conditions, the
continuous and almost uniform phase in regions where second harmonic is generated is a possible indi-
cator of coherent scattering in phase-matching conditions.
This short example simply points out one of the interest of the SHG phase, which can be obtained by
holographic SHG imaging, without even going through quantitative assessments.
9.6.2 3D-tracking of nanoparticles
In Section 9.6.1, we insisted on label-free applications. However, there seems to be a growing trend to
use labeling in SHG microscopy (Extermann et al
.
, 2009; Pantazis et al
.
, 2010). Compared to habitual
fluorescent markers, SHG markers offer the many advantages attributed to the scattering nature of sig-
nal generation. For one, they do not blink nor bleach, and thus offer stable, nonsaturating signal of
ultrafast response time, making possible observation of fast dynamics over long time periods. Another
advantage, still related to the scattering nature of signal generation, is the flexibility in the choice of the
excitation wavelength to which is linked that of the detected signal.
For the following discussion, we make a distinction between markers and (nano) probes. Markers are
exogenous contrast agents whose purpose is to reveal specific structures upon imaging. They are gener-
ally very small, for example, molecule-sized, and are used in such large quantities that they become indi-
vidually indistinguishable. Membrane potential-sensitive molecules capable of SHG (Peleg et al
.
, 1999;
Moreaux et al
.
, 2000) are an example of SHG markers, and so would be metallic nanoparticles enhancing
the signal of such markers (Campagnola et al
.
, 2001). In opposition, nanoprobes are nanostructures,
sometimes as large as a few hundreds of nanometers, which can be localized, hopefully with precision,
and tracked in time to provide functional information about the specimen. They are generally used in
much lower densities than markers, so as to be individually distinguished, localized, and tracked in both
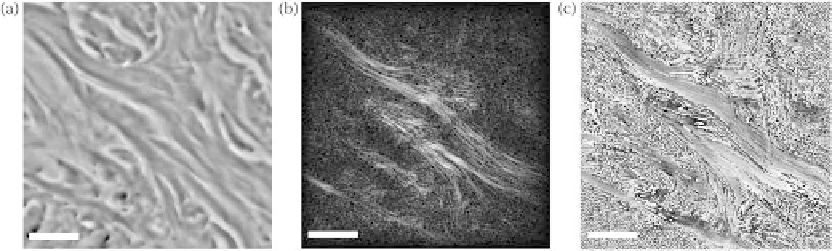