Biomedical Engineering Reference
In-Depth Information
20.7.2 B
IOMATERIALS
U
SED
FOR
I
NTESTINAL
T
ISSUE
E
NGINEERING
Although intestinal tissue engineering is currently not a viable therapeutic option for humans with
intestinal failure, a number of research groups are developing biomaterials that can be used as
scaffolds to support the engineering of intestinal constructs in small animal models. A variety of
different scaffolds have been developed to date for intestinal tissue engineering, composed of both
synthetic [77,78] and natural [79,80] polymers.
20.7.2.1
Fibrous Synthetic Polymer Scaffolds
To date, the most well-reported biomaterial used for intestinal tissue engineering has been a highly
porous, synthetic, biodegradable polymer tube fabricated from nonwoven sheets of polyglycolic
acid fi bers [77]. Stabilized polyglycolic acid fi bre-based tubes can be fabricated from polyglycolic
acid meshes by physically bonding adjacent fi bers using a spray-casting technique [81]. Rectan-
gles of polyglycolic acid mesh composed of fi bers with a diameter of approximately 12 µm were
wrapped around a 3 mm diameter Tefl on cylinder to form a tube, and the two opposing edges were
interlocked to form a seam. The Tefl on cylinder was rotated and solutions of poly(lactic-
co
-glycolic
acid) and polylactic acid dissolved in chloroform were atomized and sprayed over the rotating poly-
glycolic acid mesh. Unlike poly(lactic-
co
-glycolic acid) and polylactic acid, polyglycolic acid is
only weakly soluble in chloroform therefore the polyglycolic acid fi bers were largely unaffected by
the solvent-casting technique. The tubes were freeze-dried to remove residual solvent and removed
from the Tefl on cylinder.
The tubular scaffolds were capable of resisting relatively large compressive forces
in vitro
and
maintained their tubular structure when implanted
in vivo
. The mechanical stability of the scaffolds
was dictated by the extent of physical bonding, the polymer used to bond the polyglycolic acid fi bers,
and the processing conditions. Polylactic acid bonded tubes were more resistant to compressional forces
compared with the poly(lactic-
co
-glycolic acid) bonded tubes, probably due to the crystalline nature
of polylactic acid, making it stiffer compared with amorphous poly(lactic-
co
-glycolic acid). Simulated
degradation of the tubes in an aqueous environment
in vitro
also markedly weakened the poly(lactic-
co
-glycolic acid) bonded tubes compared with the polylactic acid bonded devices. This effect was prob-
ably caused by the increased hydrophilic nature of poly(lactic-
co
-glycolic acid), leading to the adsorbed
water acting as a plasticizer. Complete erosion of poly(lactic-
co
-glycolic acid) bonded fi bers occurred
within 11 weeks, whereas the polylactic acid bonded fi bers degraded much more slowly [81].
The ability of this type of scaffolds to support tissue growth
in vivo
has been assessed by implant-
ing them into a small animal model. Prior to implantation, the tubes were cold-gas sterilized with eth-
ylene oxide and coated with a solution of collagen type I overnight to improve the biocompatibility of
the polymer fi bers. The collagen-coated tubes were subsequently washed with Hanks' balanced salt
solution before cell seeding and implantation into the omenta of rats. In small animal models, such as
rats, scaffolds fabricated using this approach have enabled small amounts of tissue-engineered intes-
tine to be successfully grown and anastomosed to native small bowel, where it increased in size and
became lined by well differentiated neomucosa, which could be maintained for up to 36 weeks [82].
20.7.2.2
Foam Synthetic Polymer Scaffolds
In addition to fi brous scaffolds, a number of alternative scaffolds for intestinal tissue engineering
have also been developed. These include tubular scaffolds composed of PLGA foams fabricated by
a thermally induced phase separation (TIPS) process [78]. TIPS has been used to fabricate a variety
of micro- and macroporous foams. The technique involves the conversion of a single homogeneous
polymer solution made at elevated temperature into two-phase separated domains via the removal
of thermal energy. These domains are composed of a polymer-rich phase and a polymer-lean phase.
The solvent crystallizes when the polymer solution temperature is lower than the solvent freez-
ing temperature, which results in the polymer phase being expelled from the crystallization front.
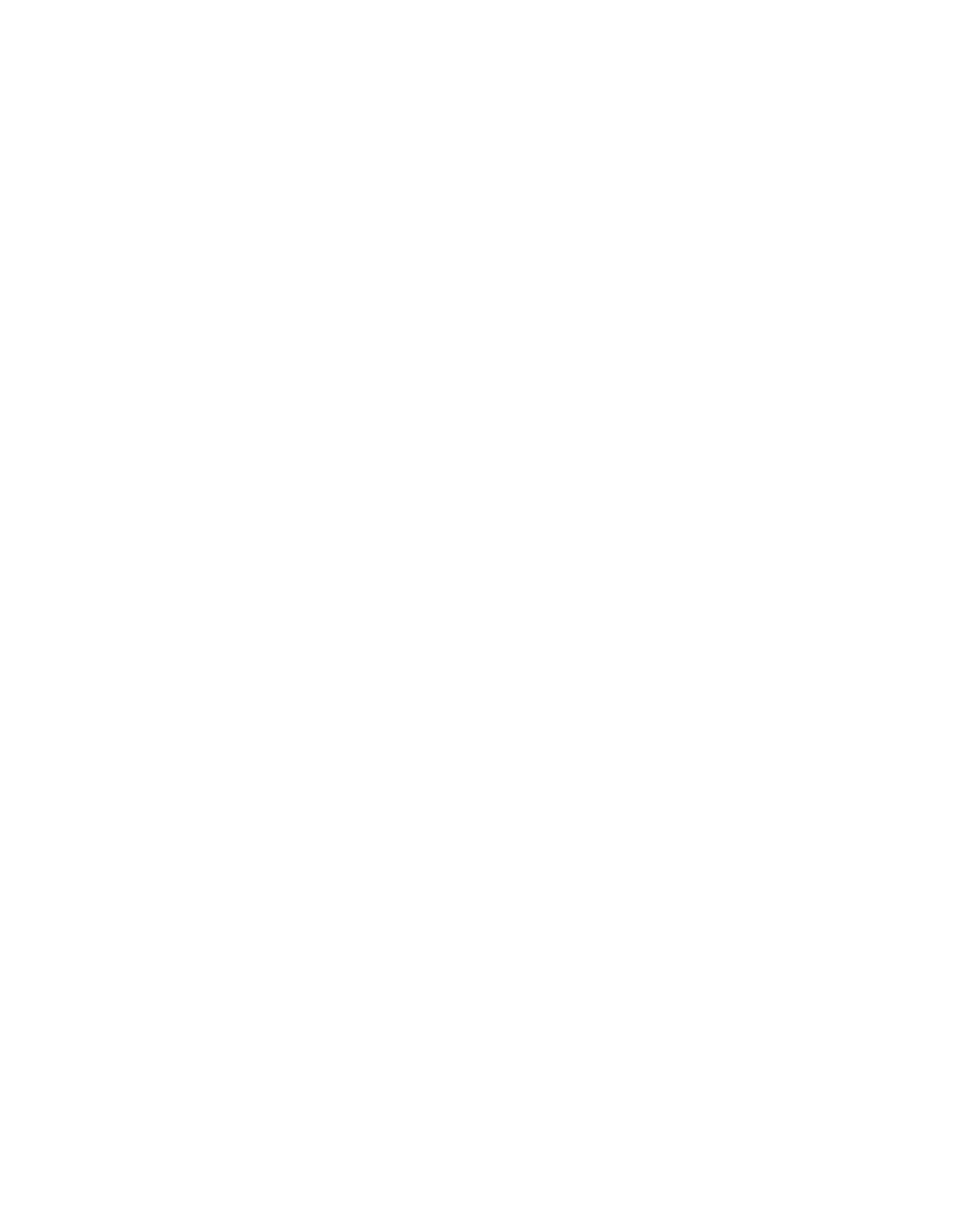