Biomedical Engineering Reference
In-Depth Information
O
CH
3
O
O
O
CH
2
C
O
CH
C
O
(CH
2
)
5
C
n
n
n
(a)
(b)
(c)
FIGURE 15.1
Structure of linear aliphatic polyesters (a) PGA, (b) PLA, and (c) PCL.
O
Catalyst
+
heat
H
3
C
CH
3
O
CH
3
O
O
O
CH
C
O
CH
C
O
CH
3
n
O
Lactide
Polylactide
FIGURE 15.2
Ring opening polymerization of lactide in the presence of a catalyst and heat.
glycolide, and caprolactone are commonly used clinically. The degradation rates vary for each
of the polymers; however, varying parameters such as molecular weight, crystallinity, copolymer,
fabrication techniques, composite formation, etc. can vary the kinetics of degradation. Poly(lactic
acid) (PLA) is one the most signifi cant members of the degradable polymer family, which can be
produced from abundant naturally occurring corns, sugars, or beetroot.
PGA belongs to the family of polyesters and is obtained through ring opening polymerization of
glycolide, and it exhibits fairly rapid degradation rates. It is a crystalline polymer and the molecular
weights can be varied by the method of synthesis. Poly(lactic) acid (PLA) is derived from lactic acid
(Figure 15.2), which is a chiral molecule and can therefore exist as d and l isomers, the l-isomer
being the biological metabolite. Hence, PLA is often referred to as PLLA, l-PLA (l-isomer), d-PLA
(d-isomer), and PDLA/dl-PLA (mixture of d and l isomers), depending on the isomeric forms used.
3
The additional methyl group renders the polymer more hydrophobic than PGA and also exhibits
slower degradation rates. PLLA is a thermoplastic material with a melting point (
T
m
) of about 180
C
and a glass transition temperature (
T
g
) higher than room temperature.
4
Thus, PLLA can be processed
into fi bers, fi lms, and blocks by techniques that are applicable for poly(ethyleneterephthalate) (PET)
and various products with superior mechanical properties.
Polymer chains can be linear, branched, or cross-linked with other chains. Polymers can be
amorphous or crystalline in nature and can typically comprise of both amorphous and crystalline
domains. The greater the crystallinity, the more ordered the structure that results in a more “tightly
bound” structure, restricting slippage between the adjacent polymer chains, which results in superior
mechanical properties. These polymers are viscoelastic in nature. Increasing levels of amorphous
domains within the polymer microstructure tend to enhance the rate of degradability and thus the
absorption of the implant. Polymers in general are affected by temperature and exhibit a typical
glass transition temperature. Polymers tend to undergo softening at a specifi c temperature and thus,
polymers used for biomedical application should have a glass transition temperature well above
the body temperature such that no distortion occurs as a result of polymer softening. Other factors
that play an important role on the properties of polymers are the conformation, confi guration, and
orientation of the molecules. PGA and PLA undergo hydrolytic degradation and are totally degrad-
able with water and carbon dioxide, its ultimate degradation products. The mechanical properties
of PLA and PGA are diverse, and literature review suggests that comparisons are diffi cult because
not only that the testing methods vary, the storage conditions, molecular weight, cyrstallinity, shape
°


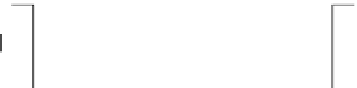

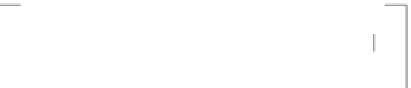












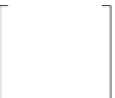
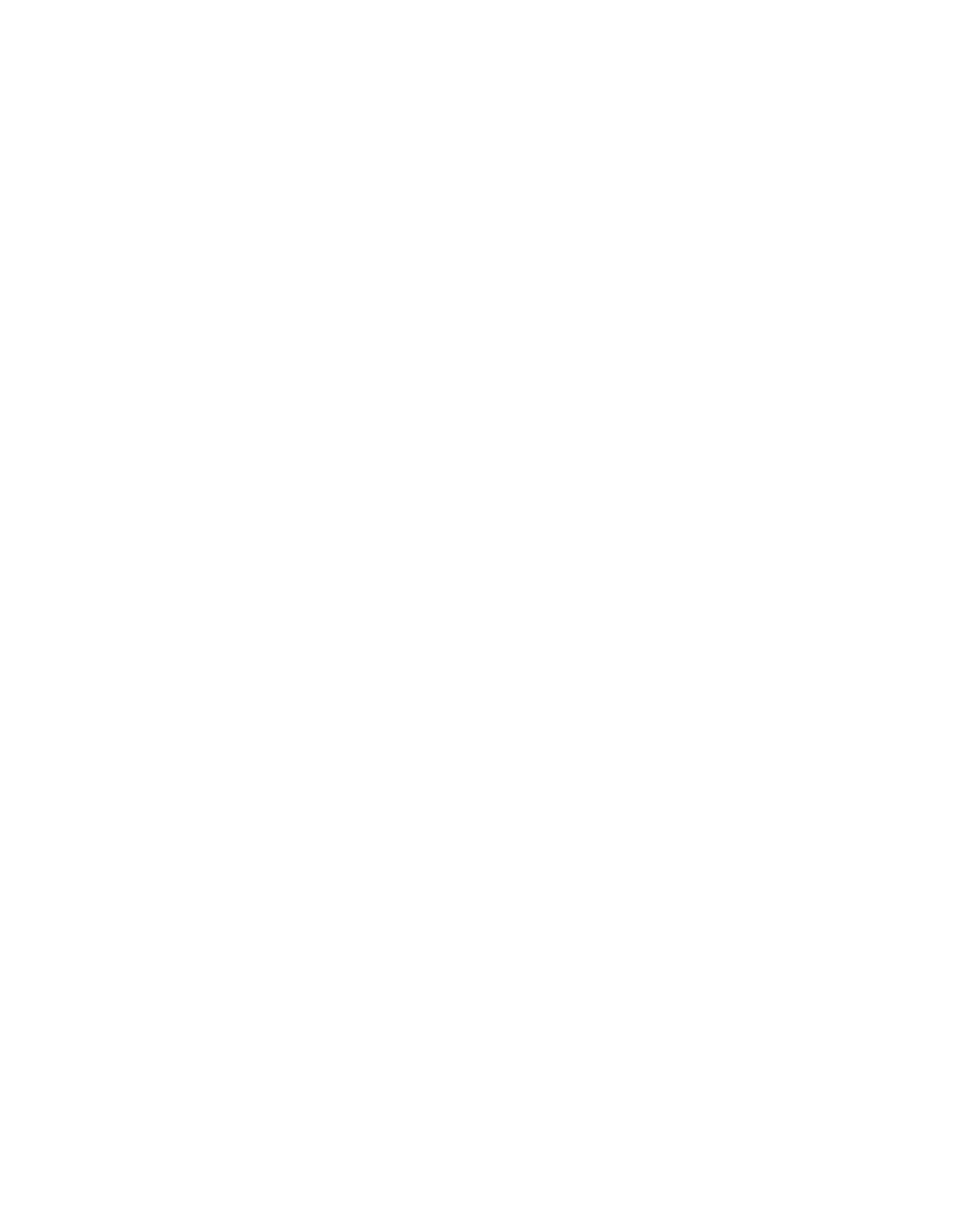





