Biomedical Engineering Reference
In-Depth Information
5.4 TISSUE ENGINEERING APPLICATIONS
BY USING ELECTROSPUN SCAFFOLDS
5.4.1 B
ONE
T
ISSUE
E
NGINEERING
Bone is a composite material made from an organic phase of collagen, glycoproteins, and gly-
cosaminoglycans (GAGs) and an inorganic phase primarily consisting of hydroxyapatite (HA)
Ca
10
(PO
4
)
6
(OH)
2
[39]. Bone serves several critical functions including muscular support, production
of blood cells and immune cells, and as a mineral reservoir to maintain electrolyte balance in the
body. Bone may be lost after trauma, cancer, fractures, periodontitis, osteoporosis, and infectious
disease, and presently there are several types and sources used as bone grafts.
Bone is the second most transplanted tissue, second to blood. Current treatment regimes for the
reconstruction or enhancement of function of damaged bone rely on autogenous or allogenic tissue
grafts. Autogenous bone, by providing an osteoconductive matrix, growth factors, and osteogenic
cells (which form the critical elements of bone repair), remains the graft material of choice despite
the associated donor site morbidity infl icted on the patient, the potential of infection at the bone
harvest site, painful surgical procedure, and the risk of injuring surrounding structures. Surgeons
are increasingly facing patient groups, which have limited supply of transplantable autologous bone
due to multiple operations, poor bone quality, and patient groups that have limitations for large bone
grafts, especially children. The continuing clinical need for improvement of existing treatments for
bone disorders ranging from congenital deformity to reconstructive surgery for tumors and trauma
repair has resulted in the search for novel approaches to skeletal reconstruction.
Bone tissue engineering aims to heal bone defects with autologous cells and tissues without the
donor site morbidity and expense associated with harvesting autogenous bone. One such strategy
involves seeding autologous osteogenic cells
in vitro
throughout a biodegradable scaffold to create
a scaffold-cell hybrid (TEC). This involves the isolation of a suitable cell population and expan-
sion to a clinically relevant size
ex vivo
, seeding the expanded population onto an appropriate 3-D
scaffold, which may be impregnated with appropriate growth factors and other chemical cues to
enhance tissue in-growth followed by further culture in a bioreactor and place
in vivo
at the requisite
tissue regeneration site. The scaffold is expected to support cell colonization, migration, growth,
and differentiation, and to guide the development of the required tissue or to act as a drug delivery
device.
Based on this background, approaches are therefore presently being investigated using scaf-
folds formed by electrospinning. Vacanti's group [40,41] used electrospun PCL membranes (average
diameter of 400
200 nm) and investigated their potential for bone tissue engineering. Mesenchy-
mal stem cells (MSCs) were seeded onto the scaffolds and cultured using a combination of static
culture and a rotating bioreactor. The MSCs differentiated into osteoblasts and migrated into the
scaffold and produced collagen. The scaffold constructs were dimensionally stable and had become
noticeably harder after 4 weeks presumably due to mineralization. In a later study, the group cul-
tured MSCs on PCL nanofi brous scaffolds in a rotating bioreactor before implanting them into the
omenta of rats. After 4 weeks
in vivo
, the scaffolds maintained their original shape with evidence of
mineralization and type I collagen expression within the graft. However, the authors failed to pres-
ent histological evidence of bone, which is comparable to the natural bone. In addition, no mechani-
cal testing on TEC was performed.
HA-polymer composite scaffolds are increasingly being used for bone tissue engineering in
an attempt to create more biomimetic constructs and to improve mechanical properties. Kim et al.
investigated HA-gelatin composite nanofi bers for bone tissue engineering [42]. Nanocrystals of
HA were well distributed throughout the electrospun fi bers (average diameter of 200-400 nm) by
employing a stepwise process, which involved initial mineralization of HA in the gelatin. Electros-
pun scaffolds were subsequently cross-linked and up to 40% HA could successfully be incorporated
using this technique. Higher amounts of HA resulted in an unstable electrospinning process and
±
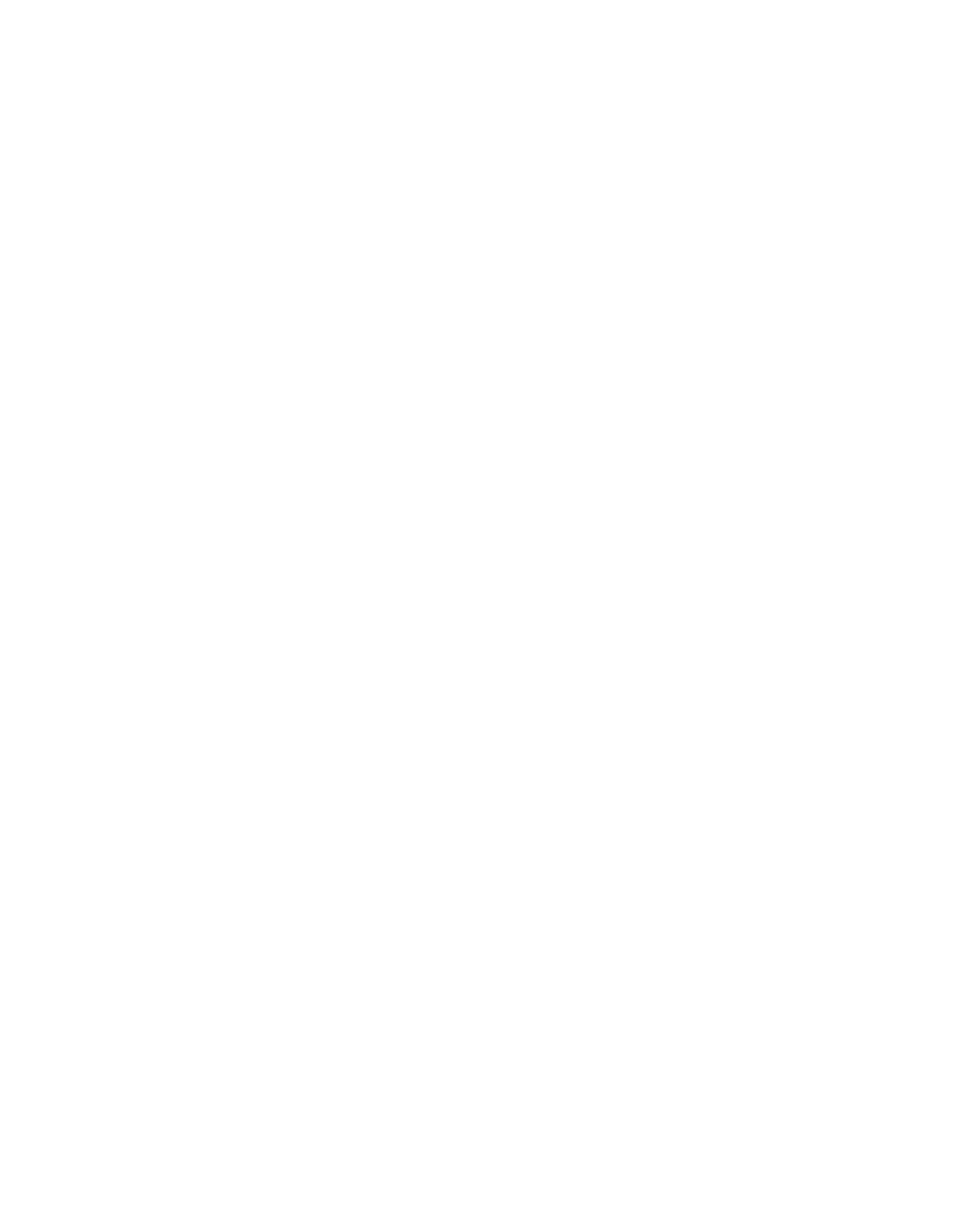