Geology Reference
In-Depth Information
Thickness of intermediate layer (
d
2
=
/
2
[
t
i
−
2
d
1
/
V
1,3
]
×
V
2,3
):
1
W end:
d
2
=
/
2
×{
0
.
060
−
25
.
2
/
790
}×
1910
=
26
.
8m
D
=
26
.
8
+
12
.
6
=
39
.
4m
E end:
d
2
=
1
/
2
×{
0
.
149
−
67
.
0
/
790
}×
1910
=
61
.
3m
D
=
33
.
5
+
61
.
3
=
94
.
8m
Stage 4 Reciprocal time interpretation (example using Geophone 8)
Reciprocal time (
t
A
+
t
B
−
t
i
):
1
W end:
t
R
=
101
+
254
−
60
=
295 ms
E end:
t
R
=
233
+
208
−
149
=
292 ms
Average
=
293 ms
Depth conversion factors at short shots (F
=
2
×
D
/
t
i
):
W end:
F
=
1310 ms
−
1
E end:
F
=
2
×
94
.
8
/
0
.
149
=
1270 ms
−
1
×
.
/
.
=
2
39
4
0
060
Example: F
at G8 (by interpolation)
=
1280 m s
−
1
Depth at G8 (
D
=
t
A
+
t
B
−
t
R
):
D
=
/
2
×
(0.174
+
0.213
−
0.293)
×
1280
=
60.2 m
1
13.2.6 Seismic refraction imaging
Traditional methods of interpreting seismic refraction data assume a layered
Earth where the layers are gently dipping (
<
10
◦
) and the velocity structure is
relatively simple. In more complex geological environments, characterised
by steeply dipping and/or discontinuous refractors or strong lateral changes
in velocity, modelling techniques that assume continuous velocity gradients
can provide more realistic results. Software packages are now available that
use finite element or finite difference approaches to automatically model
varying velocity gradients, without any prior assumptions of subsurface
structure. First-arrival picks are used to develop a best-fit velocity model
by iteratively comparing the modelled first-arrivals from different velocity
distributions with the observed data. This approach (Figure 13.10) is referred
to by some workers as refraction tomography, and the interested reader is
referred to an allied discussion on nomenclature in Section 6.5.1.
Figure 13.10 shows that perfect agreement between the observed and
modelled T-D plots was not achieved. Moreover, and in contrast to tradi-
tional methods, which require some understanding of geology as well as
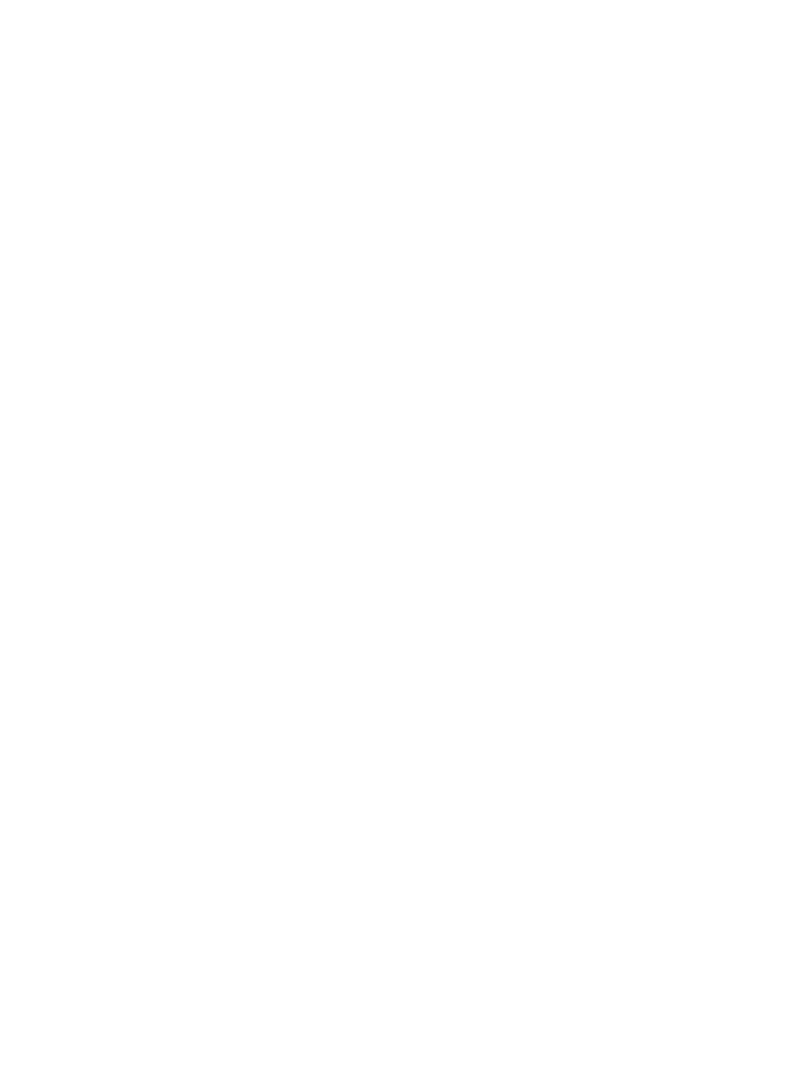
