Geoscience Reference
In-Depth Information
study period are not accompanied by commensurately large
positive trends in low-level thermal advection.
During the period 199-200 as a whole, atmospheric
circulation trends and associated changes in wind-induced
low-level thermal advection are weak in all seasons; as a
result, they are unlikely to have played a dominant role in the
overall retreat of Arctic sea ice since 199. However, Arctic
air temperatures, SSTs, and net surface downward longwave
radiation have all increased since 199 and thus collectively
provide a favorable environment for sea ice loss.
Our findings are in qualitative agreement with those of
Francis and Hunter
[2006, 200], who examined some of
the forcing factors for regional SIC variations in winter and
summer. In particular,
Francis and Hunter
[200] analyzed
satellite-derived estimates of downwelling longwave radia-
tion, low-level winds, and SSTs in relation to winter SIC
variations in the Bering and Barents seas during 199-2005.
They found that wind anomalies were the dominant forc-
ing mechanism in the Bering Sea, while a combination of
wind and SST anomalies were the main factors in the Bar-
ents Sea. In a related paper,
Francis and Hunter
[2006]
showed that downward longwave radiation was the primary
cause of summer sea ice extent variations during 199-2004
throughout the Arctic marginal ice zone. They also reported
that wind anomalies played a role in forcing summer sea ice
extent anomalies in the Barents and Chukchi seas before, but
not after, 1991. The results shown here based on SLP and
wind-induced atmospheric thermal advection trends are in
general agreement with the findings of
Francis and Hunter
[2006, 200], although we note that a direct comparison is
not possible because of the different timescales of variability
examined in the two studies (interannual and longer in the
case of Francis and Hunter and trends in our case).
In summary, our results lend additional support to the
findings of numerous studies that factors other than long-
term atmospheric circulation trends are playing a dominant
role in the overall retreat of Arctic sea ice since 199. These
include warming of the upper ocean [
Polyakov
et al.
, 2005;
Shimada
et al.
, 2006;
Stroeve and Maslowski
, 2008;
Steele
et al.
, 2008] and lower atmosphere [
Comiso
, 2003;
Serreze
et al.
, 200;
Comiso
et al.
, 2008], sea ice thinning and asso-
ciated reduction in multiyear ice fraction [
Kwok
, 200;
Roth-
rock
et al.
, 200;
Nghiem
et al.
, 200], increased oceanic
absorption of solar radiation in summer associated with the
mechanism of positive ice-albedo feedback [
Perovich
et al.
,
2007] and enhanced downwelling longwave radiative flux
due to increased water vapor, cloudiness and carbon dioxide
concentration [
Francis and Hunter
, 2006, 200]. A better
understanding of these and other processes affecting Arctic
sea ice remains an important task with relevance to future
predictions of Arctic climate change.
Acknowledgments.
We thank the two anonymous reviewers and
Editor Eric DeWeaver for constructive suggestions. We are grate-
ful to the National Snow and Ice Data Center for providing the
sea ice concentration data. Clara Deser acknowledges support from
the NSF Office of Polar Programs (ARCSS), and Haiyan Teng ac-
knowledges support from the U.S. Department of Energy under
Cooperative Agreement DE-FC02-9ER62402. The National Cen-
ter for Atmospheric Research is sponsored by the National Science
Foundation.
REFERENCES
Cavalieri, D. J., C. L. Parkinson, P. Gloersen, J. C. Comiso, and
H. J. Zwally (1999), Deriving long-term time series of sea ice
cover from satellite passive-microwave multisensor data sets,
J.
Geophys. Res., 104
, 15,803-15,814.
Comiso, J. C. (2003), Arctic warming signals from clear-sky surface
temperature satellite observations,
J. Clim.
,
16
, 3498-3510.
Comiso, J. C. (2006), Abrupt decline in the Arctic winter sea
ice cover,
Geophys. Res. Lett.
,
33
, L18504, doi:10.1029/
2006GL02341.
Comiso, J. C., C. L. Parkinson, R. Gersten, and L. Stock (2008),
Accelerated decline in the Arctic sea ice cover,
Geophys. Res.
Lett.
,
35
, L0103, doi:10.1029/200GL03192.
Deser, C. (2000), On the teleconnectivity of the “Arctic Oscilla-
tion,”
Geophys. Res. Lett.
,
27
, 9-82.
Deser, C., and H. Teng (2008), Evolution of Arctic sea ice con-
centration trends and the role of atmospheric circulation forc-
ing, 199-200,
Geophys. Res. Lett.
,
35
, L02504, doi:10.1029/
200GL032023.
Deser, C., J. E. Walsh, and M. S. Timlin (2000), Arctic sea ice vari-
ability in the context of recent atmospheric circulation trends,
J.
Clim
.,
13
, 61-633.
Fang, Z., and J. M. Wallace (1994), Arctic sea ice variability on a
timescale of weeks: Its relation to atmospheric forcing,
J. Clim.
,
7
, 189-1913.
Francis, J. A., and E. Hunter (2006), New insight into the disappear-
ing Arctic sea ice,
Eos Trans. AGU
,
87
(46), 509, doi:10.1029/
2006EO460001.
Francis, J. A., and E. Hunter (200), Drivers of declining sea ice
in the Arctic winter: A tale of two seas,
Geophys. Res. Lett.
,
34
,
L1503,
doi:10.1029/200GL030995.
Holland, M. M., C. M. Bitz, and B. Tremblay (2006), Future abrupt
reductions in the summer Arctic sea ice,
Geophys. Res. Lett.
,
33
,
L23503, doi:10.1029/2006GL028024.
Hu, A., C. Rooth, R. Bleck, and C. Deser (2002), NAO influence on
sea ice extent in the Eurasian coastal region,
Geophys. Res. Lett.
,
29
(22), 2053, doi:10.1029/2001GL014293.
Hurrell, J. W. (1995), Decadal trends in the North Atlantic Oscil-
lation: Regional temperatures and precipitation,
Science
,
269
,
66-69.
Kalnay, E., et al. (1996), The NCEP/NCAR 40-year reanalysis
project,
Bull. Am. Meteorol. Soc.
,
77
, 43-41.
Kay, J. E., T. L'Ecuyer, A. Gettelman, G. Stephens, and C. O'Dell
(2008), The contribution of cloud and radiation anomalies to the
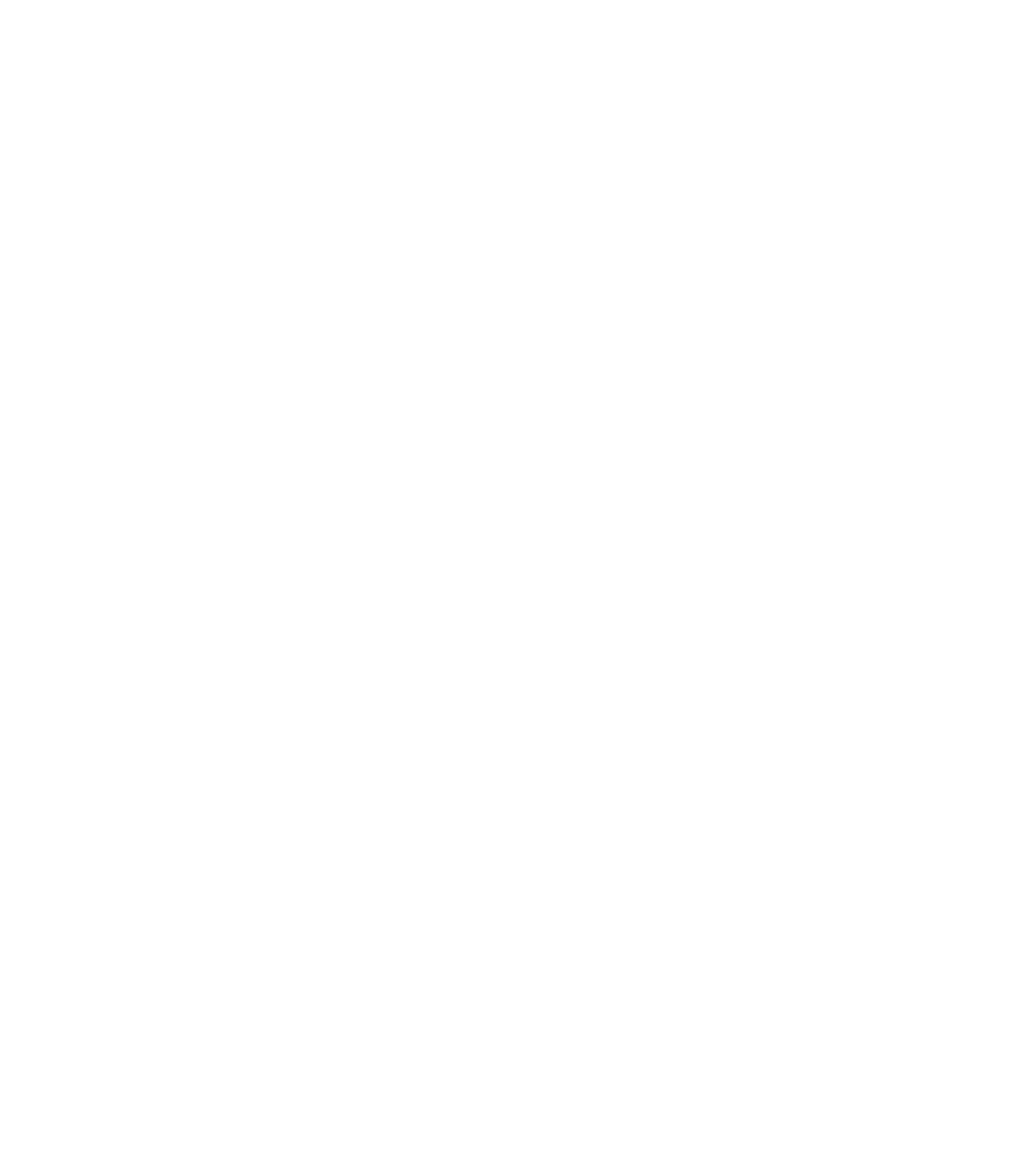
Search WWH ::

Custom Search