Geoscience Reference
In-Depth Information
occur, such as behind ventifacts or beneath sand stringers.
Prolonged periods of burial by sand are common during
seasonal or within-storm shifts in wind direction (Greeley
et al.
, 2002). At the scale of development of large ven-
tifacts, climate change occurs, influencing both the char-
acter of the rock surface through weathering, the supply of
sediments and the nature of wind flow, making estimates
of ventifact age based on present-day meteorological con-
ditions unrealistic.
Several studies have attempted to determine rates of
ventifact formation. Sharp's (1964, 1980) experimental
plot in the Coachella Valley, California, yielded a range
of abrasion rates, which varied according to time and
material hardness. It should be noted, however, that the
materials used were not rock, but softer manmade mate-
rials. Similarly, Greeley
et al.
(1984) report a relatively
wide range of inferred rates, varying from 10
−
5
of the atmosphere (the wind system) and the topography.
On a small scale, aeolian researchers recognise the influ-
ence of a range of surface roughness elements, such as
patches of coarse sediment, vegetation or boulders, which
determine how near-surface winds respond. In ventifact
fields, these fixed elements incorporate strongly varying
roughness element size (small to large boulders), density,
spacing and porosity that affect sand transport and shear
stress distribution. Field observations of ventifact forma-
tion by the author during strong winds show that the wind
and sediment environments are constantly changing. Ven-
tifacts are successively exposed, buried by sand and then
re-exposed; sand ribbons blanket and protect ventifacts
while adjacent rocks are abraded; and each ventifact af-
fects the abrasion of downstream rocks, by shielding them
or by projecting saltating grains into higher velocity layers
of the atmosphere.
At the landscape scale, the influence of topography on
the flow field becomes important (Gilbert, 1875; Laity,
1987, 1995). Flow is compressed and expanded based
on curvature and the geometrical scales of the underly-
ing surface. The topography affects flow acceleration and
deceleration, and hence the distribution of shear stress
and the pathways of sand transport. These factors have a
strong influence on ventifact scale and distribution. Stud-
ies of airflow over hills and dunes (Jackson and Hunt,
1975; Bowen and Lindley, 1977; Taylor, 1977; Lancaster,
1985; Tsoar, 1985; Mulligan, 1988; McKenna Neuman,
Lancaster and Nickling, 1997) have led to advances in
our understanding of ventifact formation. Wind velocity
increases and longer periods of abrasion near hillcrests
and in topographic saddles result in scale increases in
ventifact features (grooves or flutes) (Figure 21.18).
Some progress can be made in understanding field con-
ditions by using targets to simulate rocks, but soft materi-
als are necessary to achieve results in a reasonable period
of time (Figure 21.14). Sharp (1964, 1980) placed Lucite
rods in an exposed setting and was able to determine a
maximum height of abrasion above the ground. Bricks
showed wear after a period of 15 years, with megascop-
ically visible effects such as polish, pitting and incipient
fluting developed during an intense 10-month period of
high particle flux (Sharp, 1980). Artificial gypsum targets
of different internal consistencies and hardnesses have
been used both in the field and in the laboratory (Figure
21.14) (Bridges
et al.
, 2004; Laity and Bridges, 2009).
Field targets eroded very rapidly when placed adjacent to
rock ventifacts and developed small-scale lineations and
flutes within a few months, paralleling the direction of the
highest velocity winds and with the same orientation as
linear features on adjacent marble ventifacts.
×
10
−
1
cm/yr, determined by abrasion of a range of ma-
terials (brick, hydrocal, tuff, granite, basalt, rhyolite and
obsidian) in a wind tunnel. Based on laboratory exper-
iments, Kuenen (1960) concluded that ventifacts would
develop very slowly where only fine sand is available
(one to two centuries), quickly with medium sand (a few
dozen years) and very rapidly on stormy beaches (a few
dozen years). This latter conclusion is consistent with ob-
servations of Knight and Burningham (2003), who con-
cluded that ventifacts along the Oregon coast, abraded by
medium-grained, well-sorted sands, have a maximum age
of 60-70 years. Rates of 0.24-1.63 mm/yr were calcu-
lated for this site. Clearly, the time for ventifacts to form
in a desert setting is not yet well constrained. Further-
more, there is probably a considerable difference in the
time required to develop initial features (pitting, polish,
minor grooving) and that needed to radically change the
rock form into a mature, often wedge-shaped ventifact,
wherein a great deal of mass has been lost. In the central
Namib Desert, Selby (1977) concluded that the faceting
of boulders 10 to 50 cm in diameter would take thousands
of years to complete.
to 6
21.2.5.7
Surface controls, spatial variability, feedback
and topography
Within any given field of ventifacts, there is a wide ar-
ray of forms and surface features, even in unidirectional
winds passing over rocks of a single lithology: no two
ventifacts look alike. What can account for these differ-
ences? Unlike a wind tunnel, surfaces are not flat, grain
sizes are not uniform, there are not limitless supplies of dry
sediment, nor are there steady and uniform winds. Natural
systems are highly complicated, both from the perspective
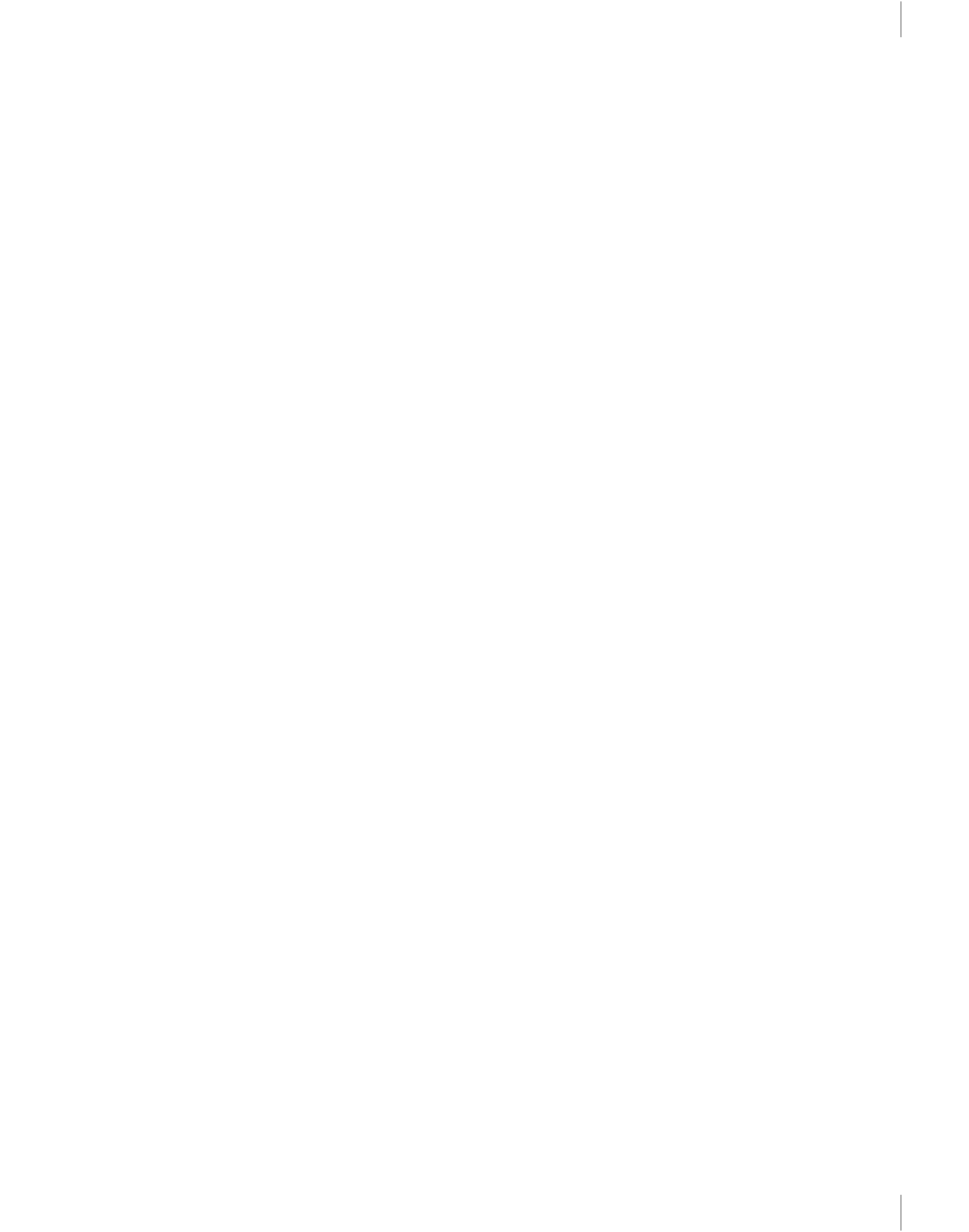