Geoscience Reference
In-Depth Information
order to determine the rates of hillslope evolution through
time. Early work in the southwestern USA by Abrahams
et al
. (1984) observed the rates of movement of a range of
particle sizes >8 mm and showed that hillslope gradient
exerted a strong control over the travel distance of parti-
cles (see also Abrahams, Parsons and Hirsh, 1985), which
travelled further with longer overland flow distances. This
work has been developed in the last 20 years through re-
lated experiments. Parsons, Abrahams and Luk (1991) and
Parsons, Abrahams and Simanton (1991) show that there
is a selective erosion of finer particles from debris-covered
hillslopes, leaving coarser particles in situ and leading to
the development of desert pavements, overlying the debris
mantle. Furthermore, Abrahams and Parsons (1991b)
show that overland flow from debris-mantled hillslopes
may subsequently be controlled by stone pavement cover
as well as stone size, which itself increases with hillslope
gradient. Finally, Parsons
et al
. (2004) postulate that sedi-
ment loss from debris-mantled desert hillslopes is strongly
controlled by the travel distance of individual particles,
with coarser particles travelling very short distances and
fine particles travelling similar distances to overland flow,
on an event basis. Such work illustrates that low-gradient,
mantled hillslopes are dominated by hydrological pro-
cesses and will evolve episodically, when sufficiently in-
tense rainfall events occur to entrain particles via overland
flow. Parsons
et al
. (2006) provided empirical support for
this interpretation for slopes at Walnut Gulch in Arizona.
Rates of talus flatiron development have been studied by
Gutierrez
et al
. (1998) and Gutierrez Elorza and Ses“eMar-
tinez (2001), who studied the rates of slope evolution un-
der flatirons in the Ebro and Almazan Basins in Northern
Spain. They concluded that scarp retreat rates were of the
order of 1-10 mm/a and corresponded well with changes
in climate over the last 30,000 years. Thus, it seems
that rates of talus-slope evolution are often controlled by
changing climates, which control the all-important supply
of moisture to the arid zone. Schmidt (2009) summarises
the important characteristics of talus-flatiron evolution in
five stages: (1) when moisture supply is high, the slope will
develop a concave profile, as supply of material is plenti-
ful. Such flatirons may then be starved of supply if aridity
increases or they may continue to develop, particularly if:
(2) highly resistant caprocks are evident to maintain sup-
ply of material that will armour the slope surface during
periods of dissection (drier periods). Some talus flatiron
slopes become highly protected as calcium carbonates are
washed into the matrix, forming a well-cemented talus
covering. (3) The thickness ratio of harder caprock strata
to softer substrate is critical, as thin overlying caprock is
insufficient to supply the flatiron surface and thus the flat-
Conversely, very thick caprock strata may oversupply the
substrate slope, which does not allow the development of
a concave hillslope profile. (4) If numerous strata of vary-
ing resistance to weathering are present, then the talus flat-
iron may not develop a fully concave profile and the talus
ledges described above may dominate. (5) Finally, trun-
cation of the toe slope by fluvial action will lead to steep-
ening of the lower profile, increasing the removal of talus
material from the flatiron base. This model can be seen as
a specific example of the ways in which different elements
of the slope system control each other by the interaction
of material supply and process rates, producing boundary
conditions across different slope units (Figure 10.1).
In certain locations, such as the semi-arid southwest-
ern USA (Schmidt and Meitz, 1996) and Northern Spain
(Gutierrez
et al
., 1998, 2001), it is clear that talus slopes
are currently evolving at significant rates, particularly
during periods of enhanced rainfall. During drier peri-
ods, or in drier locations, flatirons tend to become discon-
nected from the adjacent supply of caprock material and
will dissect, until bare rock substrates are exposed.
10.4
Conclusion
In one respect, badlands and rock slopes can be considered
to be very similar in that they are essentially bedrock sur-
faces in many drylands. However, the lack of a significant
thickness of regolith
sensu stricto
in the case of badlands
is outweighed by the typically unconsolidated nature of
the bedrock, so they tend to behave at the extreme end of
what might be expected for soil-covered landscapes. Thus,
key controls for distinguishing between the behaviour of
these stereotypical drylands must be sought elsewhere.
Such controls can be seen to be extrinsic, in the sense
of lithological, tectonic and climatic variability, and thus
operate on timescales from decadal to millions of years.
Explaining the patterns occurring within these slope sys-
tems then requires an understanding of the interactions
between processes of weathering (Chapter 6), runoff and
sediment transport (Chapter 11).
The interactions between these processes lead to the
evolution of spatial variability in slope form, and this
variability then feeds back to the ways in which differ-
ent slope elements interact with each other. In revisiting
the process-form dualism, geomorphologists need to de-
velop models of these interactions that can account for
their complexity and the ways in which they evolve over
multiple timescales. As noted also in Chapter 11, there
is an emerging set of ideas about landscape connectivity,
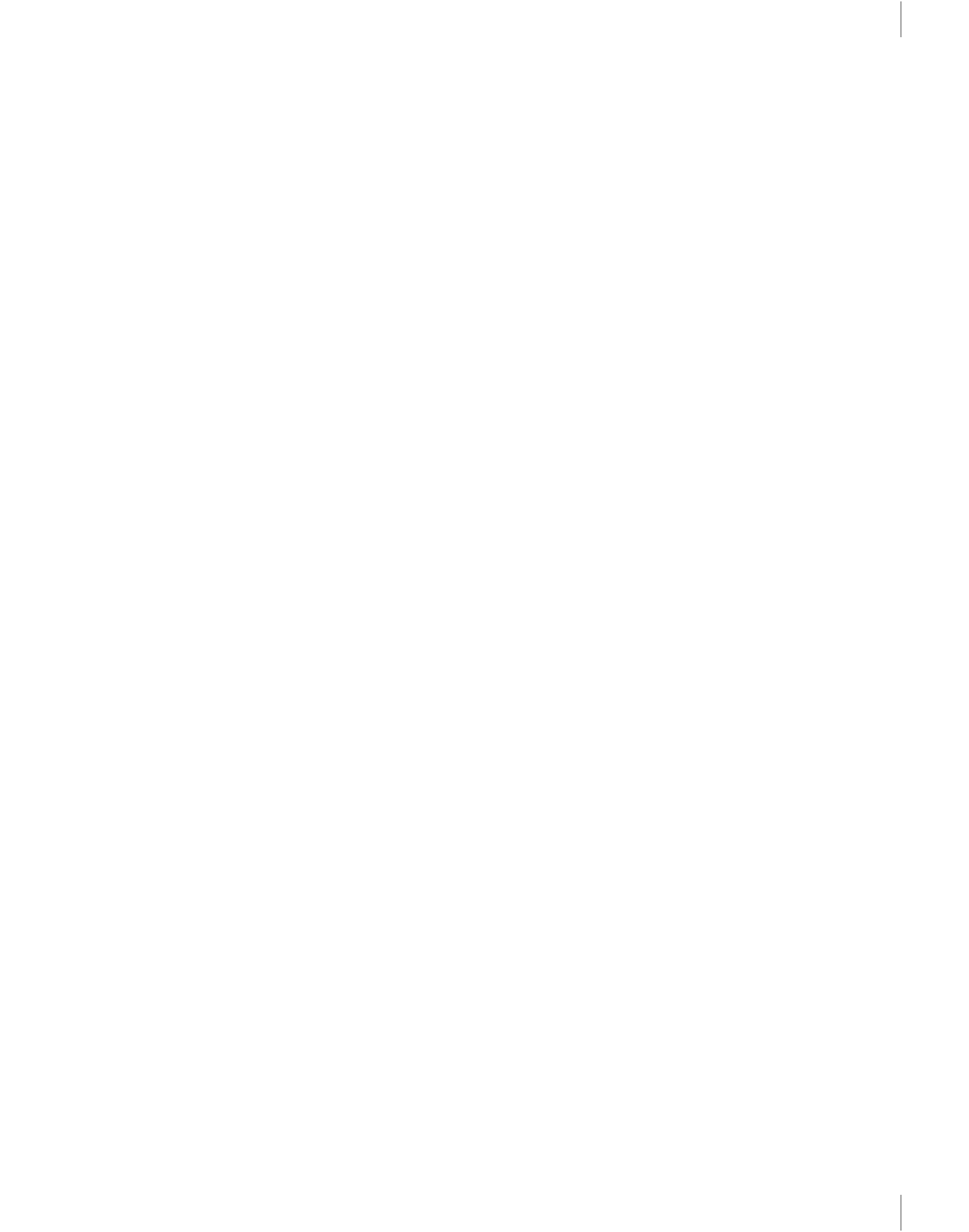