Geoscience Reference
In-Depth Information
on or under rock varnish, including lichen, fungi, bac-
teria, cyanobacteria, pollen, peptides, refractory organic
fragments, fatty acid methyl esters and amino acids from
gram-positive chemoorganotrophic bateria (Dorn, 2009).
Laudermilk (1931) and Krumbein and Jens (1981), for
example, suggested that lichen oxidise and concentrate
Mn, with algae and fungi also implicated (Bauman, 1976;
Borns
et al.
, 1980; Krumbein and Jens, 1981; Grote
and Krumbein, 1992). However, microcolonial fungi have
been shown to either coexist with or actively dissolve rock
varnish (Borns
et al.
, 1980; Staley
et al.
, 1983; Dragovich,
1993). Fungal respiration is also inhibited by the presence
of montmorillonite (Stortsky and Rem, 1967), one of the
main clay minerals in rock varnish. This may suggest in-
stead that Mn fixation is accomplished by bacteria (Dorn
and Oberlander, 1981, 1982). Dorn and Dragovich (1990)
successfully cultured nine Mn-oxidising bacteria, and the
dendritic patterns in some varnishes are similar to those
produced during Fe and Mn oxidation by
Bacillus cereus
(Billy and Cailleux, 1968). Rock varnishes are 'home'
to vast numbers of bacteria, with analyses of samples
collected from desert pavement cobbles in eastern Cali-
fornia revealing the presence of 10
8
cells of bacteria per
gram dry weight of varnish (Kuhlman
et al.
, 2005, 2006).
There are, however, two major limitations of the biogenic
model. First, the association of lithobionts with varnishes
is insufficient to explain Mn enhancement or clay cemen-
tation, as there is no unequivocal process by which these
organisms can produce rock varnish. Second, if all the
organisms observed in or on rock varnish were actively
involved in varnish genesis, rates of formation should be
several orders of magnitude higher than observed (Dorn,
2007). Where rates of varnish accumulation have been
measured, typically only one to twenty bacterial diame-
ter equivalents accumulate each thousand years (Liu and
Broecker, 2000).
The most recent model to explain varnish formation is
silica binding (Perry
et al.
, 2006). Under this model, silica
is dissolved from dust and other minerals, then gels, con-
denses and hardens, binding clay minerals, organics and
other detrital components to a rock surface. Silica binding
cannot explain varnish formation alone as the process con-
tains no mechanism for enhancing Mn levels. Dorn (2007,
2009) highlights other flaws, including the inability of the
process to explain the dominance of clay minerals in var-
nishes and the rapid speed with which silica precipitation
proceeds compared with known rates of varnish accre-
tion. As the model relies on high temperatures to 'bake'
the silica coating, it cannot explain the occurrence of sub-
surface and cold climate varnishes (e.g. Whalley, 1984;
Whalley
et al.
, 1990; Dorn and Dragovich, 1990; Dorn
In the absence of one wholly convincing hypothesis for
varnish formation, Dorn (2007) has suggested a fourth
model in which abiotic and biotic processes act in tan-
dem. Under this polygenetic model, slow-growing Mn-
and Fe-fixing bacteria colonise dust layers on rock sur-
faces. The decay of Mn- and Fe-encrusted bacterial casts
under weakly acidic conditions allows Mn and Fe to be
mobilised from the cell walls, move a few nanometres and
abiotically cement clay minerals. High-resolution trans-
mission electron microscope analyses by Dorn (1998) and
Krinsley (1998) have shown that varnishes are a product
of the nanometre-scale combination of clay minerals and
the slow accumulation and decay of Mn- and Fe-encrusted
bacterial casts. This model explains the abundance of Mn
and Fe within varnishes and, when combined with other
abiotic mechanisms (Thiagarajan and Lee, 2004), may
also account for the enhanced levels of many trace ele-
ments found in varnishes.
8.7.3
Silica glazes and iron films
Silica glaze ranges from micrometres up to a millimetre in
thickness, and comprises mostly amorphous silica (in the
form of opal) or other more crystalline silica polymorphs
such as moganite (Perry
et al.
, 2006; Dorn, 2009). It varies
in colour from charcoal black to ivory and can be almost
transparent to completely opaque and dull to highly reflec-
tive. Dorn (1998) recognises six categories of glaze. Type
I is a homogeneous deposit of amorphous silica. Type II
includes a proportion of detrital minerals, cemented by
amorphous silica. Type III is cemented dominantly by sil-
ica but incorporates 5-40 oxide weight % FeO and 5-30 %
Al
2
O
3
. This variety often has a brown appearance due to
the increased iron content and has been documented in a
variety of environments (e.g. Watchman, 1992; Curtiss,
Adams and Ghiorso, 1985; Mottershead and Pye, 1994).
Types IV and V each contain around 50 % amorphous
silica, with aluminium the main other component in the
former type and iron in the latter. Type VI glazes, domi-
nated by Al
2
O
3
, are relatively rare.
Silica glaze can form rapidly, with Curtiss, Adams
and Ghiorso (1985) describing glazes developed on his-
toric lava flows in Hawaii. It can slow rock weathering
processes and, if the glaze has penetrated sufficiently,
harden weathering rinds (Dorn, 2009). Sources of silica
are ubiquitous in deserts and include weathered bedrock,
dust, rainfall, groundwater and biological remains such
as diatom tests and plant phytoliths. Silica is also dis-
solved readily in most weathering environments. Once
transported in solution to the site of glaze formation, dis-
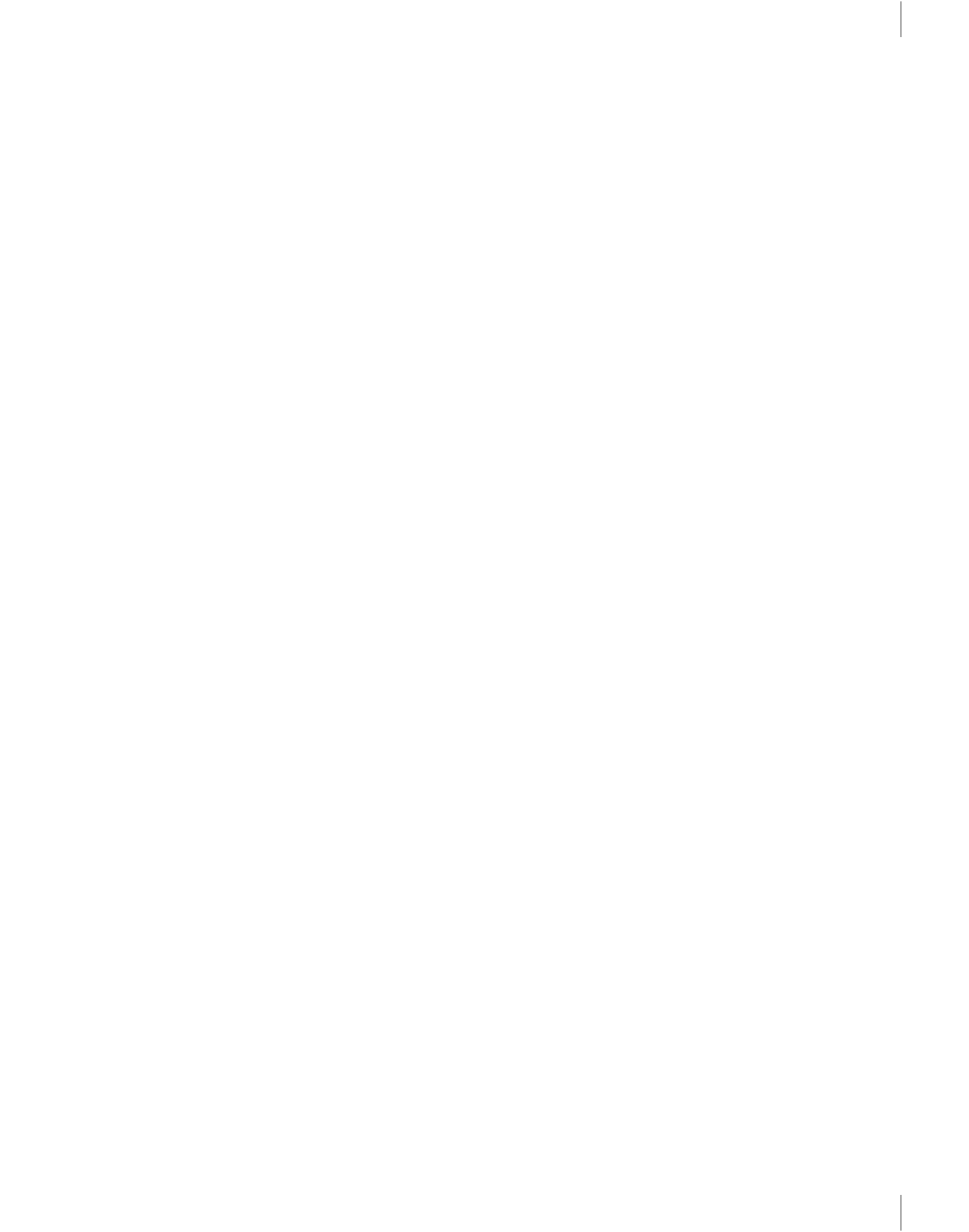