Geoscience Reference
In-Depth Information
Wheeler and Textoris, 1978) or hydrous silicates such
as magadiite (NaSi
7
O
13
(OH
3
)
profile contains two sections (Figure 8.14): an upper part,
which exhibits a columnar structure with well-ordered sil-
ica cements, and a lower part, which is weakly cemented
by poorly ordered silica (Milnes and Thiry, 1992). Semi-
continuous or lenticular pedogenic silcretes, pedogenic
silcrete 'skins', discrete nodules and veins have also been
described.
Groundwater silcretes occur as discontinuous lenses or
sheets in a range of weathered and unweathered materials
(e.g. Senior and Senior, 1972; Wopfner, 1983; Meyer and
Pena dos Reis, 1985; Thiry, Ayrault and Grisoni, 1988;
Lee and Gilkes, 2005). They develop near the ground sur-
face but have been documented at depths of up to 100 m
(Thiry, 1999; Basile-Doelsch, Meunier and Parron, 2005),
sometimes underlying or overlying pedogenic silcretes.
Groundwater silcretes exhibit simple fabrics with good
preservation of host structures. The main genetic models
arise from work in the Paris basin, where silcretes occur
in arenaceous formations and lacustrine limestones (Fig-
ure 8.12(c)). Formation in arenaceous bedrock is thought
to have taken place around zones of groundwater outflow
within valleys, with dissolved silica supplied by the chem-
ical breakdown of clay minerals in the host sediment (Fig-
ure 8.15). Silicification occurred during various phases of
Plio-Quaternary landscape incision to create a series of su-
perposed silcrete lenses. Development is thought to have
been relatively rapid, with each lens taking 30 000 years to
form (Thiry, Ayrault and Grisoni, 1988). Similar processes
have been proposed for groundwater silcrete formation in
Australia (Thiry and Milnes, 1991; Simon-Coin¸on
et al.
,
1996; Lee and Gilkes, 2005). Carbonate-hosted ground-
water silcretes may have derived their silica from over-
lying sands and soils, with silicification proceeding by
infilling of karst voids and carbonate replacement (Ribet
and Thiry, 1990; Thiry and Ben Brahim, 1997).
Drainage-line silcretes are closely related to ground-
water types but develop
within
alluvial fills in current
or former fluvial systems as opposed to within bedrock
marginal
to valley systems (Nash and Ullyott, 2007). Sil-
cretes develop at sites that are subject to seasonal wet-
ting/ drying or in zones of watertable fluctuation (Taylor
and Ruxton, 1987; McCarthy and Ellery, 1995). One
of the best examples is the Mirackina Conglomerate in
South Australia, which formed as a result of silica-rich
groundwater moving through channel alluvium within a
200 km long palaeodrainage system (Barnes and Pitt,
1976; McNally and Wilson, 1995). Few genetic mod-
els for drainage-line silicification exist. Silcretes exposed
in the Boteti River, Botswana (Figure 8.12(b)), are sug-
gested to have formed by the accumulation of clastic
and phytolithic silica from floodwater and dissolved sil-
3H
2
O), which convert to
chert or opaline silica gel when leached by dilute water
(Renaut, Tiercelin and Owen, 1986). However, this does
not imply that all silcretes are of lacustrine origin as silica
gels also form in terrestrial settings (Kaiser, 1926; Elouard
and Millot, 1959).
Environmental Eh has a complex effect upon silica sol-
ubility. Quartz placed in solutions containing ferrous iron,
for example, becomes more soluble once exposed to ox-
idising conditions (Morris and Fletcher, 1987). Evapo-
ration is significant in the development of drainage-line
(Shaw and Nash, 1998), pan/lacustrine (Stephens, 1971;
Wopfner, 1978, 1983; Milnes, Thiry and Wright, 1991)
and shallow groundwater silcretes (e.g. Lamplugh, 1907;
Waugh, 1970; Mountain, 1952) and the upper parts of pe-
dogenic silcrete profiles (Van Der Graaf, 1983; Thiry and
Millot, 1987; Webb and Golding, 1998). Silica solubility
may be reduced if adsorption on to iron or aluminium
oxides occurs. The presence of NaCl and oxides and ions
including Fe
3
+
,UO
2
+
,Mg
2
+
,Ca
2
+
,Na
2
+
and F
−
may en-
hance solubility (Dove and Rimstidt, 1994). Where sig-
nificant quantities of salts are present in solution, silica
colloids may aggregate to form hydrated opaline silica
gels (Iler, 1979). The mixing of silica-rich groundwater
with downward-percolating water rich in NaCl may pre-
cipitate silica (Frankel and Kent, 1938; Frankel, 1952;
Smale, 1973); under high salinity, chalcedony may pre-
cipitate directly (Heaney, 1993). Biogenic processes may
be of local significance for silica fixation (Shaw, Cooke
and Perry, 1990; McCarthy and Ellery, 1995).
Pedogenic silcretes typically develop on stable base-
ment or basin-marginal areas over time periods of >10
6
years (Thiry, 1978; Callen, 1983; Milnes and Thiry, 1992).
Some of the best developed profiles are in Australia and
along the south coast of South Africa (Figure 8.12(a)). It
is unlikely that many pedogenic silcretes formed under
present-day arid climates; most are instead relict features
and date from the Palaeogene or Early Neogene (Nash
and Ullyott, 2007). Thiry (1999) identifies two types of
pedogenic silcrete: those with abundant microquartz and
Ti-enrichment that lack clay minerals and iron oxides,
and more opaline 'duripans' in which clay and iron are re-
tained. Formation occurs due to cycles of downward flush-
ing of silica-bearing water followed by silica precipitation,
possibly under seasonal climates with high rates of evapo-
ration (Webb and Golding, 1998). This produces geopetal
colloform structures along water pathways, together with
glaebules and rootlets, and may be associated with evi-
dence of bioturbation (Summerfield, 1983d; Thiry
et al.
,
2006; Terry and Evans, 1994; Lee and Gilkes, 2005). Pe-
·
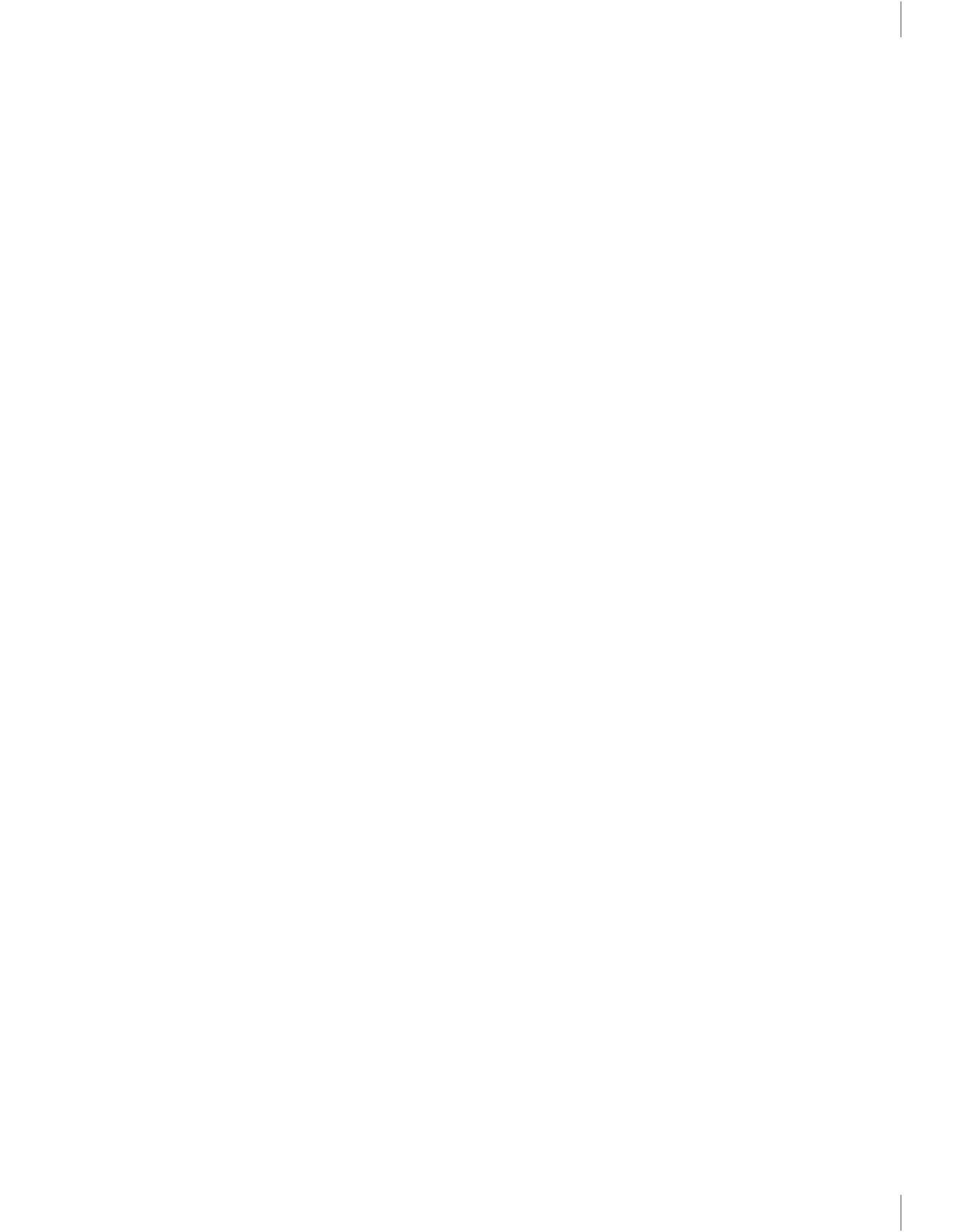