Biomedical Engineering Reference
In-Depth Information
and caprylic (8:0) acid, the latter at 20-fold excess, were dissolved in hexane and treated
with immobilized phospholipase A
2
or immobilized
R. miehei
lipase at 40 °C. The former
enzyme produced a 45% yield of the desired product, phospholipid that contained one
caprylic acyl group per molecule, and 52% of the undesired product, phospholipids
containing two caprylic acyl groups per molecule, formed as a result of acyl migration of
incorporated caprylic acyl group from the 2- to the 1-glycerol position. The product
distribution using lipase as biocatalyst was similar, and the yield of desired product was
slightly less than that achieved using phospholipase A
2
, 39%. Therefore, this result suggests
the preparation of structured phospholipids must be accompanied by selective separations
schemes, since multiple products are formed for each step of a synthesis scheme, consistent
with previous examples in the literature (Xu
et al
., 2008 ; Guo
et al
., 2005 ).
10.6 PREPARATION OF BIOSURFACTANTS VIA
FERMENTATION
In addition to employing enzymes for bio-based surfactant
via in vitro
systems, microor-
ganisms can produce bio-based surfactants
in vivo
(Kitamoto
et al
., 2009 ; Lang, 2003 ;
Muthusamy
et al
., 2008 ; Rahman and Gakpe, 2008 ; Pinzon
et al
., 2009 ). Fermentative
synthesis of bio-based surfactants, referred to as “biosurfactants,” possess many of the
advantages described above for enzymatic synthesis, particularly enhanced sustainability
(lower energy utilization, the absence of solvents, etc.). Biosurfactants are typically com-
posed of lipid, protein, and carbohydrate moieties and often are associated with cell walls
or membranes. Initial interest stemmed from their high biocompatibility and biodegrada-
bility. However, in recent years, research has shown their unique behavior in self-assembly
systems, suggesting their potential applications in biomedicine and nanotechnology
(Kitamoto
et al
., 2009). Applications of biosurfactants in food products was recently
reviewed (Nitschke and Costa, 2007).
Biosurfactants are divided into four categories: glycolipid-type, fatty acid-type (includ-
ing phospholipids, which were discussed above), lipopeptide-type, and polymer-type
(Kitamoto
et al
., 2009). Glycolipid biosurfactants include rhamnolipids, sophorolipids,
mannosylerythritol lipids, and trehalose esters (Figure 10.7). Rhamnolipids, produced
from
Pseudomonas
sp. or
Burholderia
sp. bacteria, may have a single rhamnose monosac-
charide unit, as depicted in Figure 10.7, or two, with a single product often containing a
mixture of mono- and di-rhamnolipid, with variability in acyl chain length as well. Also,
the lipophilic moiety can consist of a chain of two 2-
β
-hydroxy acids esterified together.
Sophorolipids consist of sophorose (2-O-
β
-D-glucopyranosyl,
β
-D-glucopyranose) with
an ether linkage between its reducing end and the hydroxyl of an
-1- hydroxyl fatty
acyl group of 16-18 carbons. The carboxylic moiety of the fatty acid component can be
free, or can form an ester bond with the 4
ω
or
ω
′
hydroxyl of sophorose, as depicted in
Figure 10.7. Also, the 6 and/or 6
hydroxyl groups of the sophorose unit can be acylated,
as depicted in Figure 10.7, or free. Typically, the product obtained from fermentation is a
mixture of both types.
Rhamnolipids are secondary metabolites produced during a stationary growth stage,
often when deprived of a nutrient such as nitrogen, and often utilize long-chain lipophilic or
non-traditional carbon-energy sources, such as seed oils and alkanes (Pinzon
et al
., 2009 ).
Their recovery from the fermentation can be challenging due to the frequent occurrence of
foaming (Pinzon
et al
., 2009 ).
′
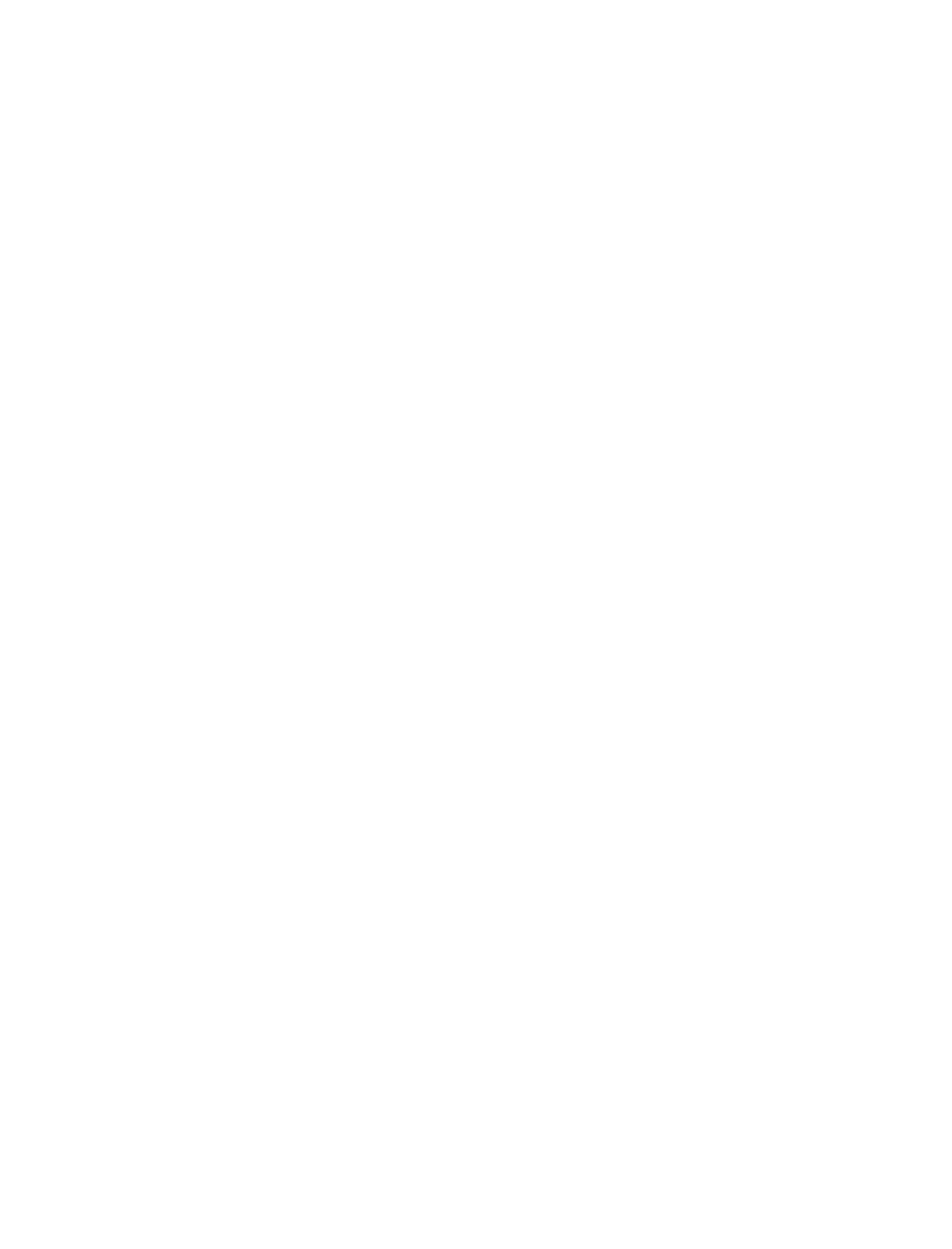



Search WWH ::

Custom Search