Biomedical Engineering Reference
In-Depth Information
the concentration of aqueous IPA (Shah and Venkatesan, 1989). This process can be
carried out at room temperature and atmospheric pressure, reducing the energy
consumption for oil refining. Because of the large difference between the boiling points
of the solvent and lipids, solvent removal from refined oil and extract stream can be easily
achieved by evaporation or distillation at relatively low temperatures (less than 80 °C),
which could be further reduced to 55 °C by performing the solvent separation under
moderate vacuum. A continuous deacidification system using aqueous ethanol (6% water)
reduced FFA content of corn oil from 3.5% to less than 0.3% (Pina and Meirelles, 2000).
The TAG loss was less than 5%, which is significantly lower than the values reported for
alkali or physical refining of corn oil. Selective solvent extraction is a potential alternative
partial deacidification process. However, use of a second solvent such as ethanol or
isopropyl alcohol besides the hexane used for extraction of oils from oilseeds may require
higher capital and energy costs.
4.4.2.5
Membrane refining
There have been a number of attempts to utilize ultrafiltration and nanofiltration for
deacidification of oils. The molecular weights of FFAs and TAGs are less than 300 Da and
larger than 800 Da, respectively. Ideally, hydrophobic nanofiltration membranes with a
molecular weight cut-off around 500-600 Da could be used to separate FFAs from TAG.
However, in practice the difference in molecular weights of FFAs and TAGs is too narrow
for utilization of nanofiltration membranes to separate these two groups of compounds.
There have been some efforts to use non-porous denser polymeric membranes for oil
refining. FFA rejection of non-porous membranes was 8-27% when sunflower oil used for
the experiments (Subramanian
et al
., 2001). In another study, simultaneous desolventizing
and deacidification by using membrane technology was examined. About 40% reduction in
FFA content and greater than 50% savings in the energy needed for hexane evaporation have
been reported while processing a model system containing 20% soybean oil with 2% FFA
(Raman
et al
., 1996). It appears that the selectivity of the current membranes is not high
enough for industrial adoption of membrane deacidification process.
4.4.2.6
Esterification of FFA
Identification of microbial lipases capable of synthesizing a TAG molecule from a fatty acid
and glycerol has kindled the research on the utilization of these enzymes for oil deacidification.
The approach was to esterify FFAs present in crude oil with added glycerol or mono or
diacylglycerides (DAG) to produce TAGs. Indeed, the FFA content of rice bran oil was
reduced from 30 to 3.6% by esterification of the FFA with added glycerol using a 1,3-specific
lipase (Mucor miehei) (Bhattacharyya and Bhattacharyya, 1987). Esterification of crude
palm olein FFAs with DAG using a lipase from
Pseudomonas fluorescens
has also been
reported (Bhosle and Subramanian, 2005). The extent of FFA esterification was higher when
glycerol was replaced with DAG. This result was attributed to the better solubility of DAG in
oil. Enzymatic esterification of FFAs with monoglycerides has also been demonstrated in the
laboratory. Although enzymatic esterification of FFAs neutralizes oil, increases TAG
concentrations, minimizes oil loss and produces a high quality product, low conversion rates
and the high cost of enzymes remain an issue for commercialization of this process.
The esterification of FFA can also be done chemically. The reaction is performed at high
temperature, usually over 200 °C, with or without a catalyst. Zinc and tin chlorides are
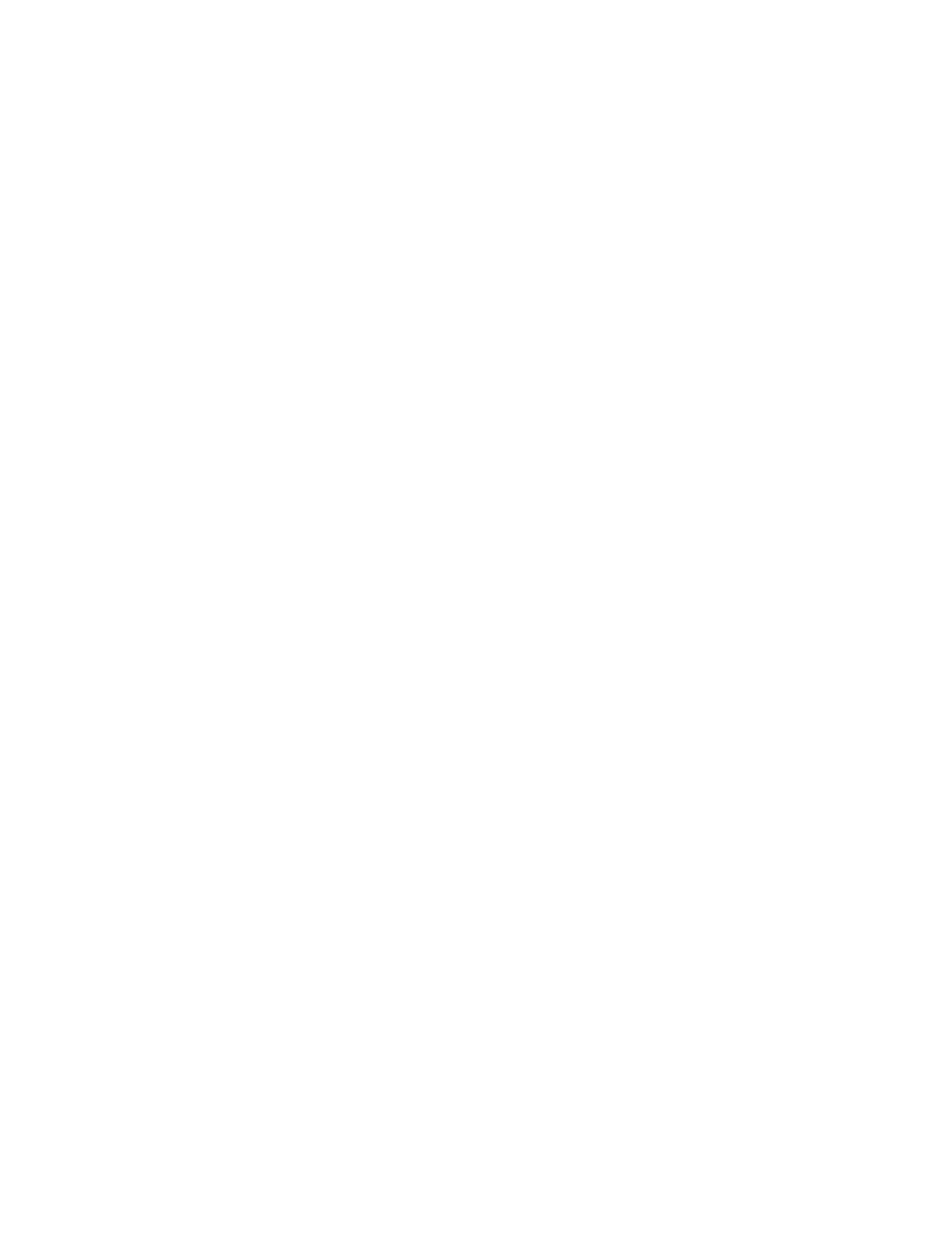



Search WWH ::

Custom Search