Biomedical Engineering Reference
In-Depth Information
Russin and co-workers (2007) reported that by decreasing the average particle diameter
of the starting raw material (i.e. soy flour) from 223.4 to 89.5 μm increased total solids
recovery from 23 to 32%, while protein recovery increased from 40 to 52%. Final protein
content (i.e. purity) of the soy protein isolates was, however, not significantly impacted by
average particle size. The results demonstrate that protein recovery can be significantly
increased by decreasing the average particle size of the starting raw material (i.e. defatted
soy flour), without having any detrimental effect on the purity of the final soy protein isolate.
In the processing of pulse proteins, protein contents of fractions extracted using different
techniques have been variable, probably due to differences in the processing conditions used
(Boye
et al
., 2010a). Chakraborty and co-workers (1979) extracted pulse proteins at pH 8.5
(30 min, 40 °C) followed by precipitation at pH 4.5 and obtained isolates containing
88.3-90.5% protein for chickpea, pea, Great Northern bean, lentil protein, lima bean, and
mung bean isolates. Paredes-López and co-workers (1991) used similar conditions and
obtained a chickpea isolate containing 84.8% protein. In the latter study, chickpea protein
isolates were obtained from defatted chickpea flour using an initial aqueous extraction at
10% (w/v) solution and pH 8.5, followed by precipitation at pH 4.5, centrifugation and
freeze drying. In other studies, de-hulled faba bean flour and pea flour were dispersed
in water (1:5 w/v ratio, pH 9, 20 min, room temperature) followed by centrifugation and
protein precipitation from the supernatant (pH 4, room temperature, 20 min). The extracts
obtained contained 81.2 and 84.9% protein, respectively (Fernández-Quintela
et al
., 1997 ).
In addition to yield and purity, conditions used during processing (pH, temperature,
extraction times, solvents) can modify protein structure and affect protein thermal stability
and functionality. These conditions, therefore, need to be properly selected taking the final
product application into consideration.
3.5.1 Protein characterization
3.5.1.1
Biochemical methods
Slab gel electrophoresis
Electrophoresis is widely applied for protein separation and characterization. The technique
involves the separation of proteins on the basis of their mobility in an electric field. Proteins
move with different speeds depending on their charge, shape and size. Polyacrylamide
gels (PAG), which are formed by the copolymerization of acrylamide monomers with a
cross-linking agent such as bisacrylamide, are generally used as the matrix for electropho-
resis (Dunn, 1989). Different types of gels containing a linear or non-linear concentration
gradient of polyacrylamide can be used to optimize protein separation (Dunn, 1989).
A range of electrophoretic techniques are available which can separate proteins on the
basis of their properties, such as size, net charge and relative hydrophobicity. However,
the most used electrophoresis techniques for the characterization of food and bioproduct
proteins are: native PAGE, SDS-PAGE, immunoelectrophoresis and isoelectric focusing.
Protein detection for these different methods is often done by staining with Coomassie
Brilliant Blue in a fixative solution or, after fixation, with silver, gold and fluorescence or
chemiluminescence probes. More detailed information on protein staining can be found
elsewhere (Amersham, 1999 a).
Electrophoresis separation can be monodimensional (1D) or bidimensional (2D). In 1D
electrophoresis, proteins are separated in one dimension on the basis of only one property,
such as charge (isoelectric point) or size. 2D electrophoresis combines the 1D electrophoresis
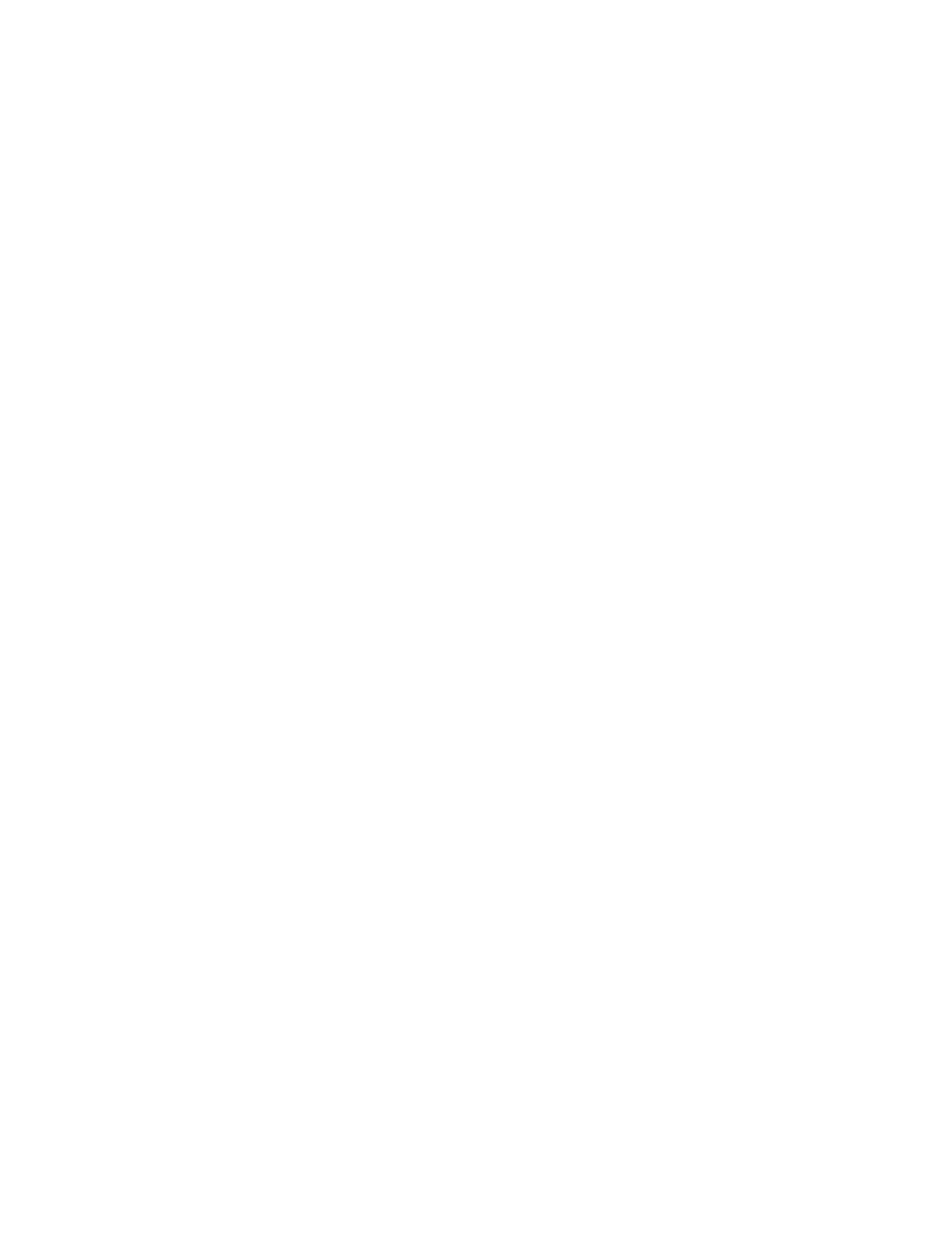



Search WWH ::

Custom Search