Geology Reference
In-Depth Information
10
-12
-10
-15
per second, or 100,000 to
10 million times slower! Nevertheless,
the correspondence between the types
of crystal behaviour and rock fabrics
seen in these experimentally deformed
materials and those characterising
natural rock deformation suggests
that they do provide a useful guide.
crystal returns to its original shape,
but if the stress is maintained for too
long, or is increased, the distortion may
become permanent and the material
begins to behave in a ductile manner.
(lithology, grain size etc.), the tempera-
ture and lithostatic pressure, the pore-
fluid pressure, and the differential stress.
Sedimentary rocks near the surface
will usually deform by means of
grain
boundary
sliding, since the grain
boundaries are the weakest part of the
rock. In unconsolidated material such
as beach sand, for example, this behav-
iour is obvious, as the grains will merely
roll past each other under stress (
granu-
lar flow
). In sedimentary material where
the grains are cemented together, and in
crystalline rocks, small fractures along
the grain boundaries will enable the
Permanent strain
Ductile deformation, or
creep
, in rocks
is achieved by means of several different
mechanisms that produce changes in
shape (strain) within the rock that are
not recoverable. There are a number of
factors that determine which mecha-
nism is chosen in a particular case;
these include the nature of the rock
Elastic behaviour
Temporary or elastic strain in a crystal-
line solid is achieved by distortion of
the
crystal lattice
, which is the molecu-
lar framework of the crystal (Figure
4.12A). When the stress is removed, the
crystal
axis
twins
crystal
axis
glide
planes
C
twin gliding
B
dislocation gliding
A
elastic distortion
crystal
axis
crystal
axis
crystal
axis
E
diffusion/ solution creep
D
rotation + gliding
Figure 4.12
Deformation mechanisms in crystalline rocks.
A.
Elastic distortion of the crystal lattice.
B.
Gliding on dislocation planes (dislocation creep) within a crystal; the orientation of the crystal axes is
unchanged.
C.
Gliding on twin planes within a crystal; the orientation of the crystal axes is unchanged
except within the twins.
D.
Gliding on dislocation planes combined with rotation to produce a more
favourable alignment with the strain axes; this produces a new orientation of the crystal axes.
E.
With
diffusion creep or solution creep, there is mass transfer within the crystal from areas of high stress
to areas of low stress; the crystal axes are unchanged.
F.
A combination of pressure solution and
recrystallisation causes favourably oriented crystals to expand and unfavourably oriented crystals
to contract, since crystals with their strongest axes perpendicular to the stress will be preferentially
favoured. Note that mechanisms B-F will all potentially produce a shape orientation fabric, whereas
only D and F can produce a crystal orientation fabric.
F
pressure solution + recrystallisation
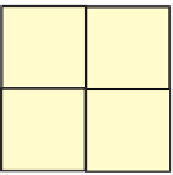
















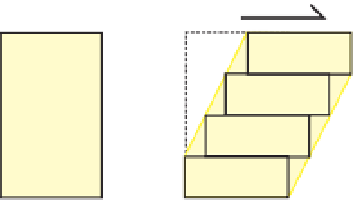
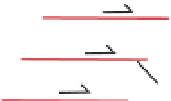



















































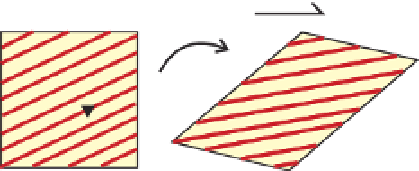





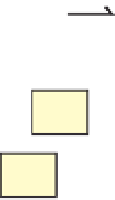
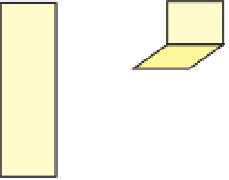








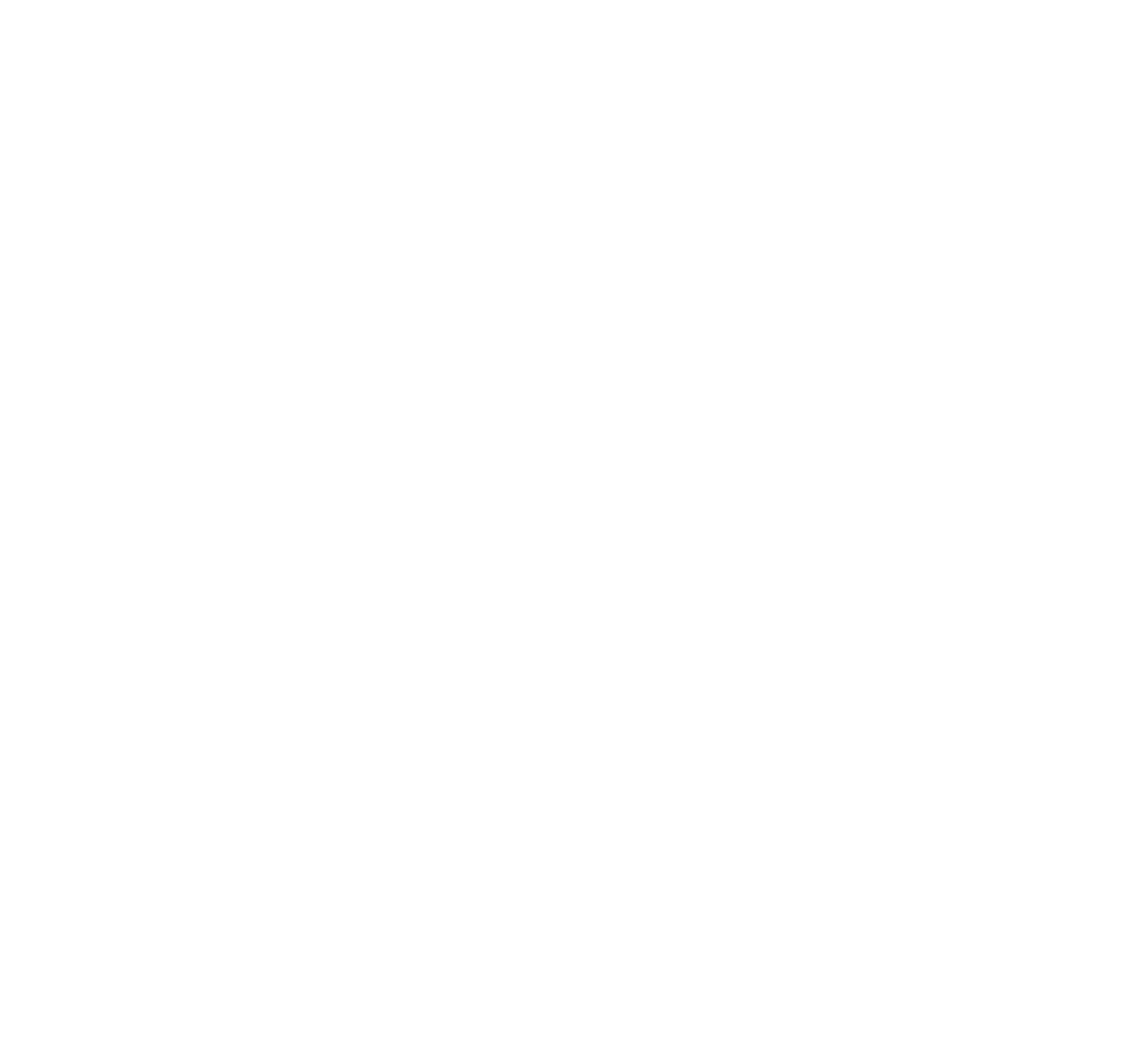
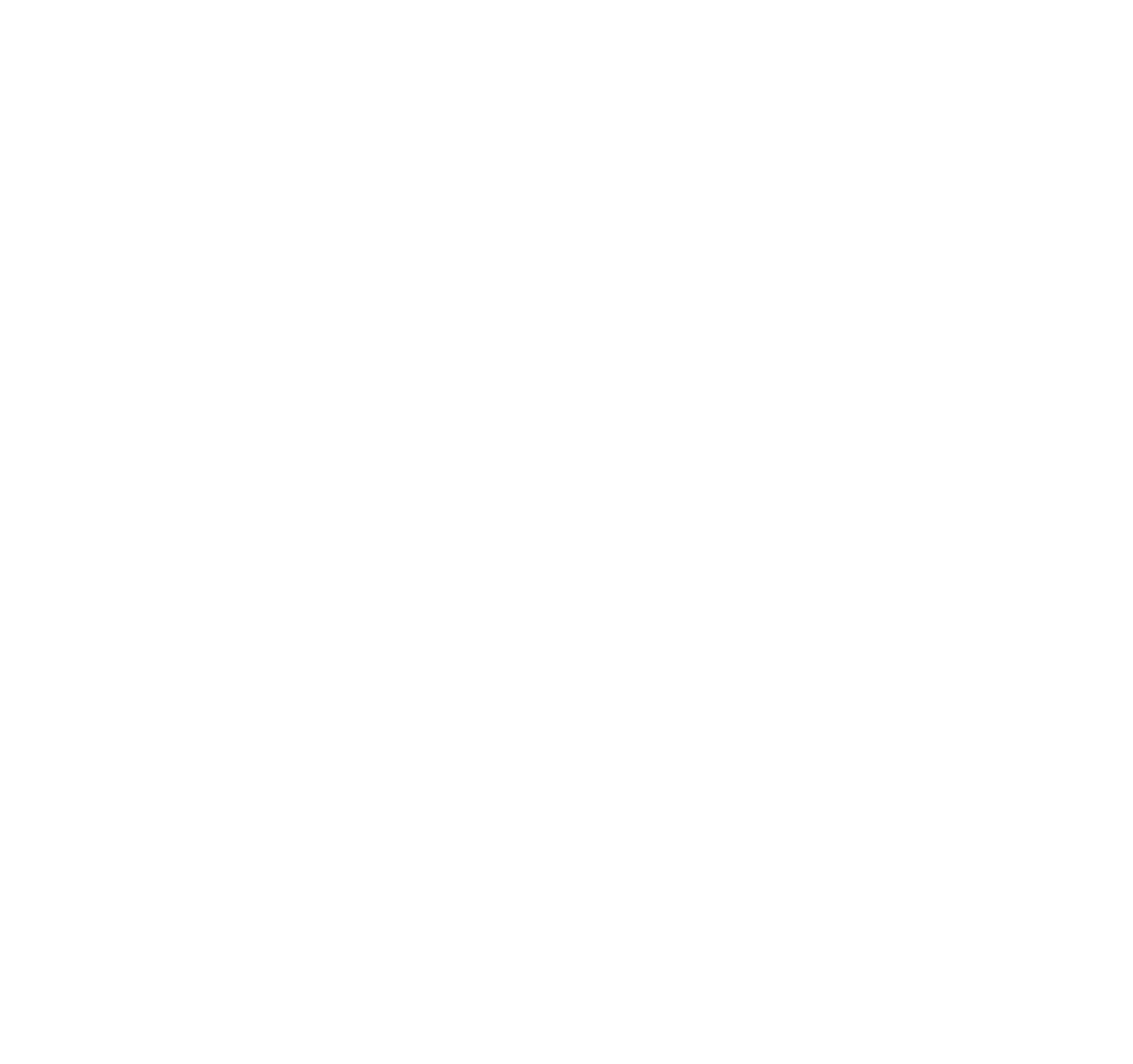

























Search WWH ::

Custom Search