Image Processing Reference
In-Depth Information
Also, because magnetization is always partially recovered at the time of appli-
cation of the following saturation pulses, multiple excitation in overlapping
regions may result in unwanted interactions and the refocusing of spoiled
magnetization. This leads to suboptimal signal suppression in the overlapping
areas. Therefore, to assure complete spoiling of undesired signals, the OVS
concept can be combined with volume preselection.
For removal of spurious lipid signals, additional methods can be used such
as lipid nulling by means of preparation pulses (37) or using spectral-spatial
selective pulses when both only the desired areas and the desired frequency
bandwidth are excited at a time (34,38,39). Lipid signals can also be removed
by postprocessing methods (40-42). The main disadvantage of lipid nulling is
the T1 weighting of all metabolite signals in the spectra. Concerning spectral-
spatial pulses, their length depends on the maximum achievable slew rate, result-
ing in rather long duration of the pulses, small excitation bandwidth, and also
limited minimum echo time of the sequence. Generally, suppression of unwanted
lipid resonances becomes less critical when long echo times are used, because
T2-relaxation time of lipids is much shorter than that of metabolites. However,
when quantitative analysis of signals from metabolites having short T2 is desired,
volume preselected CSI sequence and outer-volume suppression are the methods
of choice.
11.3.3
R
ECONSTRUCTION
OF
CSI D
ATA
In Subsection 11.3.1 the effect of 1-D phase encoding was demonstrated. In this
subsection the generalized reconstruction of 3-D CSI will be reviewed and the
concept of the point-spread function introduced.
Let us assume a 3-D CSI sequence with the phase-encoding gradients
G
=
(G
x
, G
y
, G
z
) applied along the orthogonal coordinate system described by unit
x y z
directions are incremented in N
x
, N
y
, and N
z
steps of sizes
vectors
i
,
i
, and
i
. As shown in
Figure 11.13b
, gradient strengths along all three
G
z
.
Introducing corresponding increment indexes l, m, and n, then
G
l,m,n
, representing
the discrete value of the resulting applied gradient, equals
∆
G
x
,
∆
G
y
, and
∆
G
l,m,n
=
l
∆
G
x
i
x
+
m
∆
G
y
i
y
+
n
∆
G
z
i
z
l
=
−
N
x
/2 ….
(
N
x
/2)
−
1; m, n correspondingly
(11.6)
Each gradient combination can be associated with the discrete vector
k
l,m,n
τ
∫
k
=
γ
G
(t)dt
=
l
∆
k
i
+
m
∆
k
i
+
n
∆
k
i
(11.7)
l,m,n
l,m,n
xx
y
y
zz
o
The integral in Equation 11.7 assumes the general case of the time-dependent
gradient
G
. In this case, Equation 11.2 and Equation 11.3 have to be changed
correspondingly.
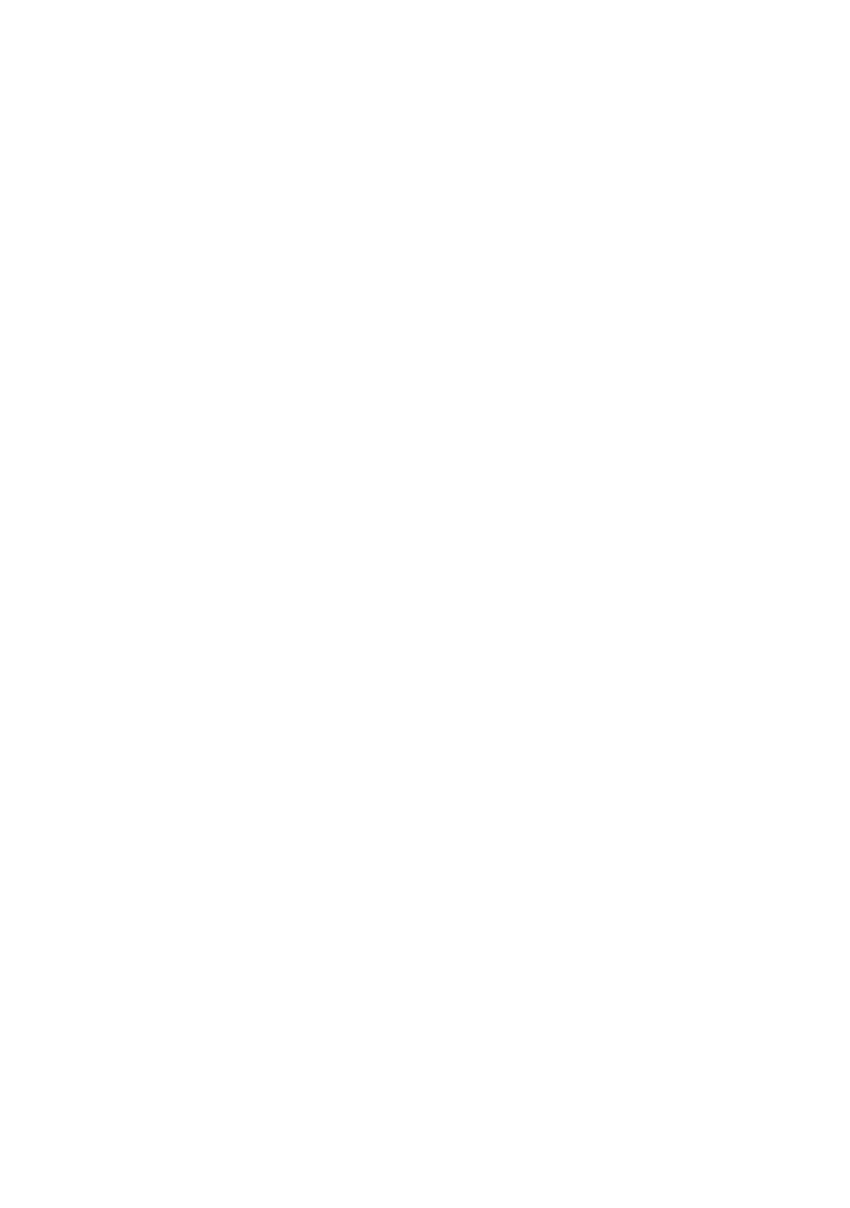





































Search WWH ::

Custom Search