Geology Reference
In-Depth Information
scenario suggests that erosion rates experience
much greater oscillations in the northern, drier,
glacial areas than in the southern, wetter, fluvial
regions, but that the integrated rates over
multiple climate cycles may be comparable.
Certainly more research is needed to document
how erosion rates change during glacial times and
whether different segments of an orogen erode at
different rates under glacial versus non-glacial
climates. Concurrently, we need to understand
better how snowline gradients change in different
climate regimes, how storm tracks vary and how
moisture enters into ranges as climate changes,
what geomorphic thresholds modulate erosion
fluxes, and how to best interpret cooling ages in
terms of erosion rates as topography evolves.
Hillslope Angles versus Rainfall
45
4
glacial
realm
40
2000
0
0
Distance
50
35
30
25
Mean angle = 46 -0.005 (MAR)
r
2
= 0.94
20
Greater Himalayan Series (GHS)
Tethyan Series & GHS
GHS & Lesser Himalayan Series
15
1
2
3
4
Mean Annual Rainfall (m/yr)
Fig. 10.44
Correlations of mean annual rainfall with
hillslope angles in the central Himalaya.
As rainfall decreases from 3.3 to 1.5 m/yr, mean hillslope
angles increase about 10
°
. These changes appear
independent of rock type, but the rainfall-hillslope
correlation does not persist through the glacial realm.
Inset shows rainfall gradients over three years. Data
from the Marsyandi catchment in central Nepal.
Modified after Gabet
et al
. (2004a).
Dynamic topography
Dynamic topography represents topographic
anomalies that result from subsurface loads or lith-
ospheric stresses that raise or depress topographic
surfaces with respect to the geoid. Commonly,
these are long-wavelength anomalies that may
span hundreds of kilometers, and their effects are
usually subtle when viewed at decadal scales or
short wavelengths. Overall, dynamic topography
produces some spectacular geomorphic effects
that were not well recognized until recently.
Geophysical studies in the southern Sierra
Nevada of California have identified a “drip”
of high-density, mantle lithosphere that has
detached from beneath the Sierras and is sinking
into the asthenosphere (Zandt, 2003). The
downward pull of this excess mass is causing
subsidence of the overlying Central Valley
(Fig. 10.45) (Ducea and Saleeby, 1998; Saleeby
and Foster, 2004). As sediments continue to
aggrade in the Central Valley, they onlap the
subsiding bedrock and create a classic “drowned”
topography, although, in this case, the drowning
is caused by sediment aggradation, rather than
by relative sea-level rise. The zone of onlap
corresponds spatially with the approximate extent
of the “drip” and includes topographic anomalies,
such as Tule Lake (Fig. 10.45A), which lies in a
closed depression in what was originally a
longitudinally drained valley that fed San Francisco
pore pressures on steep slopes are driven above
a local threshold for stability by storms (Gabet
et al
., 2004b). So, as the climate becomes increas-
ingly dry, slopes might get progressively steeper,
such that the critical pore pressure could be
reached with lesser rainfall amounts. An increase
in average slope angles in the Marsyandi occurs
concurrently with the decrease in rainfall (Gabet
et al
., 2004a) (Fig. 10.44).
A (perhaps) final possibility is that glacial
erosion in the dry realm during colder climates
compensates for slower erosion there during
interglacial climates, such as today's. Ice-age
glacial cover was much greater north of the
Himalayan crest than south of it. Whereas most
of the southern Himalayan flank is dominated
by fluvial erosion and hillslope processes even
during glacial times, expanded glaciers and
periglacial weathering in the north during
glacial times may compensate for the reduced
erosion that occurs when climate is warmer and
glaciers are retracted, as is the case today. This










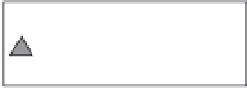







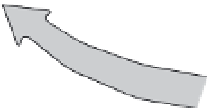

















































































