Geology Reference
In-Depth Information
rate of mountain building waned, erosion was
proposed to overtake deformation rates, thereby
causing a gradual reduction in the residual
topography (see Fig. 1.2).
A third approach to landscape development
was proposed by Hack (1975), who suggested
that, when rates of deformation and rates of ero-
sion are sustained for long intervals, landscapes
will come into a sort of balance or dynamic
equilibrium. Given the finite strength of rocks,
Hack realized that topography could not increase
without limit, even if rates of tectonic forcing
persisted for very long periods. At some point,
as topography grew and grew, the relief on
hillslopes would create forces exceeding the
rock strength, and they would collapse. With
continued uplift of the bedrock, additional slope
failures would limit the height that the topogra-
phy could attain. Eventually, the topography
would enter into a rough steady state or a
dynamic equilibrium. Consequently, in this
model, rates of mountain building and rates of
erosion would come into a long-term balance
(see Fig. 1.2). Unlike the models of Davis or
Penck, there is no need for rates of deformation
to become negligible, or even to wane at all.
Similarly, after attaining a maximum sustained
topography, there is no need for the landscape
to “evolve.” Instead, it simply fluctuates around
an equilibrium topography until such time as
the rates of tectonic forcing change.
One might wonder whether any of these
theories are applicable to real landscapes. One
way to consider this problem is to compare the
time scales of deformation and of geomorphic
responses. For example, an earthquake is a very
impulsive event that “instantaneously” creates
topographic change, say in the form of a fault
scarp. If the time between earthquakes is long
relative to the time it takes geomorphic
processes to cause significant topographic
evolution, the scarp will be degraded before
the next earthquake. This topographic change
could be thought of as a small-scale version
of a Davisian scheme for landscape evolution.
On the other hand, if the recurrence interval
between earthquakes is short, then the scarp
will be continuously refreshed and will not
degrade in a predictable fashion toward a
Catchment Components
Interfluves
Hillslopes
Channel Network
Drainage Divide
(catchment boundary)
Fig. 1.3
Geomorphic elements of a drainage basin.
Diverse geomorphic components of a catchment have a
hierarchy of inertia to change, with the channel network
as most responsive, and the drainage divide as least
responsive.
low-relief surface. In fact, some balance might
be expected to develop between the renewal of
the scarp by faulting and erosion of it during
interseismic periods. Thus, even though the total
displacement grows through time, the scarp
itself might attain a “steady-state” shape.
It is also useful to consider the response times
or inertia of different elements in a geomorphic
system. Consider, for example, a drainage basin
in a mountain belt (Fig. 1.3). The basin consists
of river channels, hillslopes, the crests of inter-
fluves, and the drainage divide that defines the
shape of the catchment. Some of these elements
will respond more rapidly to changes imposed
on them than will others. Suppose that, near its
outlet from the mountains, this basin is bounded
by a normal fault. How will the various basin
elements respond to displacement on that fault?
The channel that crosses the fault will “see” the
displacement first. The local base level will have
fallen abruptly, causing the river gradient to
steepen locally and erosion of the channel
to intensify. At this point, all other positions in
the catchment will have been undisturbed by
the faulting event, so the faulting elicits no initial
geomorphic response. Subsequently, a wave of
erosion of the channel bed can be expected to




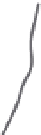












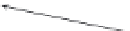

































