Geography Reference
In-Depth Information
Figure 4.12. (Left) Local
topography with estimated classes of
HAND model for site C1 in the Asu
catchment, central Amazonia.
Triangles show average water table.
Classes are (a) waterlogged, (b)
ecotone, (c) slope and (d) plateau.
After Nobre et al.(
2011
). (Right)
Comparison of the HAND model
classes (pixels) with field assessment
(circles).
the field scale. Peschke et al.(
1999
) mapped the type of
runoff generation mechanism for different catchment
states in a small German catchment based on many years
of field experience. They then developed an expert
system, known as FLAB, that estimates the dominant
runoff mechanism, such as Hortonian (infiltration excess)
overland flow and saturation excess overland flow, inter-
flow (shallow subsurface flow), recharge and storage for a
given point in the landscape and a given event size. The
expert system is based on macro-scale rules, rather than
on laboratory-scale equations and therefore implicitly
accounts for the natural co-evolution of soils, landscape
and vegetation in a catchment. Scherrer and Naef (
2003
)
developed a similar prediction scheme for dominant run-
off processes and storage based on irrigation experiments,
where the focus was on the vertical soil structure and
topography. The scheme was tested in a number of stud-
ies (e.g., Naef et al.,
2002
; Scherrer et al.,
2007
; Helleb-
rand et al.,
2011
). As the scheme requires detailed
information about the soil profile, a simplified scheme
was proposed by Schmocker-Fackel et al.(
2007
) that uses
regionally available data rather than data collected during
reconnaissance field trips. The field-based information on
runoff mechanisms, however, tends to be more informa-
tive than the regional information. Much information can
be obtained during field trips by reading the landscape
(see
Chapter 3
).
Markart et al.(2004) went a step further and explicitly
exploited the co-evolution of soils and vegetation in
Alpine catchments. The idea behind this approach is that,
depending on the soil characteristics and soil moisture
dynamics, different plant communities will be encoun-
tered, which in turn will affect soil structure and the soil
moisture state. Markart et al. therefore exploited both
vegetation type and soil characteristics for inferring the
relative contributions of surface and subsurface runoff.
The indicators used include vegetation species, land use,
soil texture, drainage density and slope, which can be
assessed during reconnaissance field trips. The maps so
obtained could then be used to assist in parameterising
distributed models in ungauged catchments (e.g., Rogger
et al.,
2012b
). Both Scherrer and Naef (
2003
) and Mar-
kart et al.(2004) focused on shallow subsurface flows.
For deeper subsurface flows an assessment of the hydro-
geology is needed. Rogger et al.(
2012a
) proposed a
framework to identify dominant hydrogeological pro-
cesses within a catchment based on orthophotos, geo-
logical maps, hydrogeological maps, digital terrain
models, maps of unconsolidated sediments, runoff spot
gauging and the use of hydrogeological expert judgement
during field trips. The method of Rogger et al. distin-
guished five hydrogeological runoff process classes: deep
groundwater flow, shallow groundwater flow, interflow,
surface runoff on rocks, glaciers or saturated areas and
karstic areas (
Figure 4.13
). Such hydrogeological classifi-
cation can provide qualitative information for rainfall
-
runoff modelling about the storage capacities and the
depth of subsurface flows in ungauged catchments. Add-
itional information on the subsurface can be provided by
tracer data, in particular if they can be related to climate
and catchment characteristics (see
Section 4.3.2
) they can
be used in ungauged catchments.
4.5 Informing predictions of runoff
in ungauged basins
In the previous sections we have discussed the process
realism of runoff predictions with respect to flow paths
and storage and the kinds of information that can contrib-
ute to achieve this realism in ungauged basins. The most
important item is an understanding of the flow system of
the catchments of interest, in particular how deep the flow
paths are in different parts of the catchment, how well they
are connected, and how long the water stays in the catch-
ment. Key to this understanding is a hydrogeological con-
cept, based on the geological history and architecture of the
catchment.
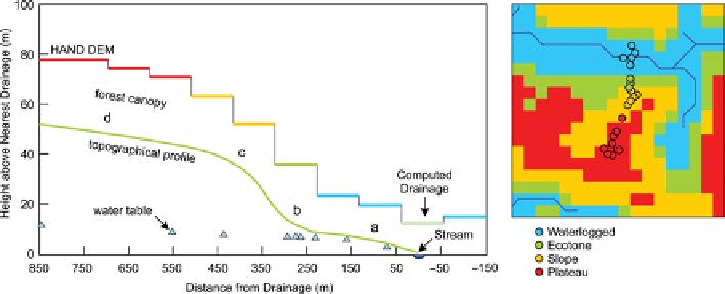
Search WWH ::

Custom Search