Geography Reference
In-Depth Information
Figure 2.2. Soil
-
ecosystem
evolution along a climate gradient in
the south-western USA. From
Rasmussen (
2008
).
understood. Jefferson et al.(
2010
) present an example of
this in Oregon, USA, i.e., the net effects of co-evolution
and hydrology in the basalt landscape in the Oregon Cas-
cade Range. They showed how dominant runoff processes
differ in catchments formed on lava flows that have different
ages. Younger catchments exhibit subdued response to pre-
cipitation as most water infiltrates and percolates into the
permeable bedrock, recharging deep aquifers that generate
runoff through permanent springs. Older catchments, on the
other hand, have deeper soils with shallow clay layers that
create impeding layers, blocking infiltrated water from
recharging the aquifers and instead cause shallow subsurface
flow that quickly enters the channel network during rain
events. At the landscape scale, this change in dominant flow
processes causes more incision and a higher drainage dens-
ity. This, in a nutshell, is the process of co-evolution as it
applies to hydrology. Humans often play an important role in
altering landscape characteristics, wherein their activities in
some environments depend on water availability and through
their actions they also affect the water availability (Sivapalan
et al.,
2012
). The co-evolution of processes that have led to
landscape patterns and their relationship to temporal and
spatial patterns of hydrological response is a key to a broader
understanding of hydrological response, including that under
human-induced changes.
Because of the coupling between different processes across
many spatial and temporal scales, catchments are complex
systems (Rihani,
2002
; Raupach
, 2005
; Kumar,
2007
;Blöschl
and Merz,
2010
). These are systems with a large number of
strongly interdependent variables at many space and time
scales. Complex systems are different from simple systems
that contain a small number of dimensions only, such as
simple mechanical systems. Simple systems are predictable
in a deterministic sense and have limited complexity. Com-
plex systems are also different from random systems with a
very large number of dimensions, such as a gas. Random
systems are predictable in a statistical sense and the tracers
may be complex at the molecular scale, but as one goes up in
scale the variability averages out (Dooge,
1986
).
A simple illustration of the difference between simple
systems and complex systems is presented in
Figure 2.3
,in
relation to flood processes and flood estimation in Austria.
The left panel on
Figure 2.3
illustrates a traditional reduction-
ist way of relating precipitation and catchment time scales
to the flood time scale. The flood response time is the sum
of storm duration and catchment response time. But in real
catchments these three time scales are not independent
(
Figure 2.3
, right panel) and the interplay amongst them
can be interpreted differently at different time scales, from
hours to millennia. The events that produce the maximum
annual floods are those for which the storm duration is close
to the concentration time of the catchment, because the
catchment-response time scales filter the distribution of all
storms to produce the distribution of flood-producing storms.
This is the reasoning behind the rational method for flood
estimation and it applies at the event scale. At the seasonal
time scale, flood characteristics tend to be closely related to
the seasonal water balance and, conversely, runoff event
types affect the seasonal water balance through rainfall and
snowmelt. At the time scale of decades, however, the flow
paths as well as soil moisture affect erosion during floods
and soil evolution (modulated by differences in geology),
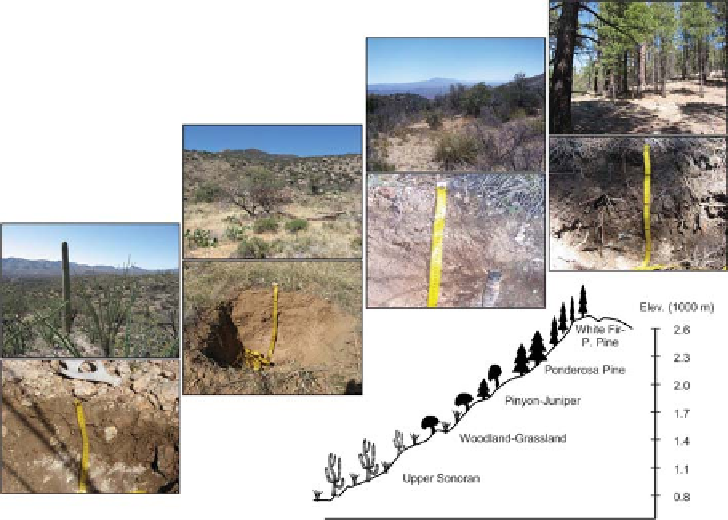
Search WWH ::

Custom Search