Environmental Engineering Reference
In-Depth Information
constitutive equation describing plane II can be written as
de
=
C
t
d
log
σ
−
u
a
+
C
m
d
log
u
a
−
u
w
(13.85)
where:
C
t
=
slope of the intersection line of plane II with the
void ratio versus log
σ
−
u
a
plane and
C
m
=
slope of the intersection line of plane II with the
void ratio versus log
u
a
−
u
w
plane.
Line
b
, associated with plane II, is assumed to be parallel
to line
a
, which joins planes I and III (Fig. 13.28b). This
assumption is required in order to construct the intersection
lines of plane II on the extreme planes. The above approxi-
mation is also applicable to the unloading surface of the void
ratio constitutive surface (Ho, 1988). The character of the
water content constitutive surface is not fully understood.
Further insight into the relationship between the consti-
tutive surfaces can be obtained through examination of the
Pham-Fredlund (2005) volume-mass model, which is based
on a series of reasonable and verifiable assumptions. The
volume-mass model commences with a saturated soil and
allows a variety of stress state changes to be applied to the soil.
(a)
(b)
13.6 PHAM-FREDLUND VOLUME-MASS
CONSTITUTIVE MODEL (2011a)
Figure 13.28
Logarithmic form for void ratio constitutive sur-
face for loading conditions: (a) void ratio constitutive surface
for loading when plotted using log-log scale for state variables;
(b) approximation of void ratio constitutive surface on log-log plot
(from Ho, 1988).
There is no single, unique relationship between overall vol-
ume change (and phase volume changes) for all possible
stress paths that can be applied to an unsaturated soil. This
is even true for isotropically loaded soils. Total volume
changes and water volume changes in an unsaturated soil
constitute independent processes. Changes in net normal
stresses can produce behavior that is independent of changes
in soil suction. The Pham-Fredlund (2011a) volume-mass
change model simulates mechanical behavioral changes (i.e.,
stress-strain behavior) and capillary behavioral changes (i.e.,
adsorption-desorption behavior) (Pham, 2005; Fredlund and
Pham, 2006; Pham and Fredlund, 2011a). Modeling the
effect of soil suction changes is quite different from mod-
eling the effect of net normal stress changes in an unsatu-
rated soil.
Deformations associated with the overall soil mass are
directly related to changes in the volume of voids (or pores)
in the soil. Total volume change and water volume change
result in changes in the volume and shape of the soil pores.
The “shape” of a pore is referred to as an “open pore diam-
eter” (or “neck pore diameter”) or a “body pore diameter,”
according to Haines (1930) capillary theory. The open pore
diameter is related to the air-entry value of the pore defined
by the drying curve. The body pore diameter on the wetting
curve is referred to as the water-entry value of the pore.
regions defined by planes I and III. Therefore, the void
ratio constitutive equation describing plane I or III can be
written in a general form as
de
=
C
t
d
log
σ
−
u
a
+
C
m
d
log
u
a
−
u
w
(13.83)
The water content constitutive equation describing plane
I or III can be written as
=
D
t
d
log
σ
−
u
a
+
D
m
d
log
u
a
−
u
w
(13.84)
dw
Plane II represents a transition zone between planes I
and III. This plane intersects both the void ratio versus
log
σ
−
u
a
plane and the void ratio versus log
u
a
−
u
w
plane. These intersection lines define the slope of plane II,
namely, the
C
t
and
C
m
indices. Two indices are required
to describe void ratio changes when the stress state variable
changes occur within the region of plane II. The
C
t
and
C
m
indices are evaluated from the
C
t
and
C
m
indices. The




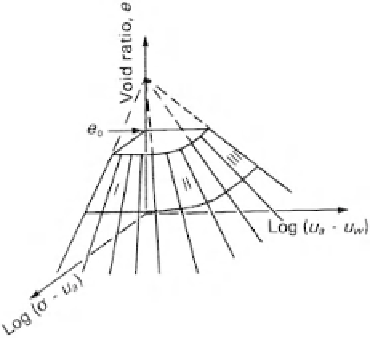
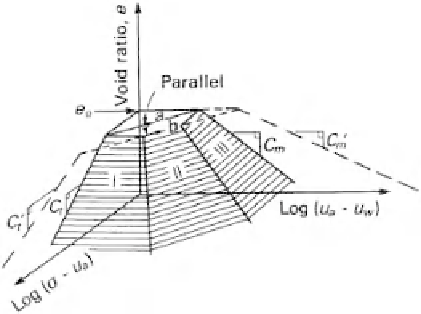





Search WWH ::

Custom Search