Environmental Engineering Reference
In-Depth Information
10
−
4
10
−
5
10
−
6
10
−
7
10
−
8
10
−
9
10
−
10
10
−
11
10
−
12
10
−
13
10
−
14
10
−
15
10
−
16
10
−
17
Experimental data
Fredlund and Xing (1994)
van Genuchten-Burdine (1980)
van Genuchten-Mualem (1980)
Brooks and Corey (1964)
Campbell (1974)
Vapor permeability
10
6
0.1
1
10
100
1000
10,000
100,000
Soil suction, kPa
Figure 7.19
Liquid water, vapor, and overall permeability coefficients with respect to soil
suction for silty sand (after Ebrahimi-Birang et al., 2004).
occupies a portion of the soil pores. The liquid water
permeability starts to decline as air enters the soil pore
space. When the liquid water coefficient of permeability
k
w
reaches a value near the residual water content conditions,
the vapor coefficient of permeability
k
v
becomes a max-
imum value. However, the
k
w
at residual conditions still
appears to be dominant over the
k
v
term.
The intersection point of liquid water and water vapor
coefficients of permeability is the point after which the vapor
permeability coefficient begins to be dominant. The intersec-
tion point provides an estimation of the minimum value for
the liquid water coefficient of permeability. Ebrahimi-Birang
et al.(2004) suggested that a reasonable lower limit for the
water coefficient of permeability is 1
while satisfying the conservation of mass. The conservation
of mass for steady-state seepage of an incompressible fluid
dictates that the flux into an element must equal the flux out
of an element. In other words, the net flux must be zero at
any point in the soil mass.
The slow movement of water through soil is commonly
referred to as seepage or percolation. Seepage analyses may
form an important part of studies related to slope stability,
groundwater contamination control, earth dam design, and
many other geotechnical engineering applications. Seepage
analyses involve the computation of the rate and direction of
water flow and the pore-water pressure distributions within
the flow regime.
Seepage analyses have historically focused on the flow of
water in the saturated zone. However, water from the atmo-
sphere (precipitation) passes through the unsaturated soil
zone on its way to becoming part of the groundwater system.
Seepage through dams used to be analyzed by considering
only the zone below an empirically computed line of seep-
age or phreatic line (Casagrande, 1937). With our present
understanding of the physics of flow through the unsaturated
soil zone, along with the available computational ability of
the computer, it is possible to model the continuous flow
of water between the saturated and unsaturated zones, as
shown in Fig. 7.20a (Freeze, 1971; Papagiannakis and Fred-
lund, 1984). Another example is shown in Fig. 7.20b, which
illustrates the effect of infiltration and evaporation on pore-
water pressures above the phreatic surface within a slope.
Generally the infiltration and evaporation processes are con-
sidered as transient processes which respond to a moisture
flux boundary condition. Steady-state water flux through the
unsaturated zone of the slope requires a constant water flux
across the ground surface boundary.
Water flow through unsaturated soils is governed by the
same flow law as through saturated soils (i.e., Darcy's law).
10
−
14
m/s.
×
7.4 PARTIAL DIFFERENTIAL EQUATIONS
FOR STEADY-STATE WATER FLOW
Geotechnical engineers are often interested in knowing the
direction and quantity of flow through porous media as well
as the pore-water pressures (or hydraulic heads). Seepage
problems can be categorized as steady-state or unsteady-state
flow analyses. The hydraulic head and the coefficient of per-
meability at any point in the soil mass remain constant with
respect to time for steady-state analyses. For an unsteady-
state analysis, the hydraulic head (and possibly the coefficient
of permeability) changes with respect to time. Changes in
hydraulic head are usually in response to a change in the
boundary conditions with respect to time. The formulation of
steady-state flow analyses are considered first, followed by
unsteady-state flow.
The quantity of flow of an incompressible fluid such as
water is expressed in terms of a flux
q
. Flux is equal to
a flow rate
v
multiplied by a cross-sectional area
A
.The
governing partial differential seepage equations are derived




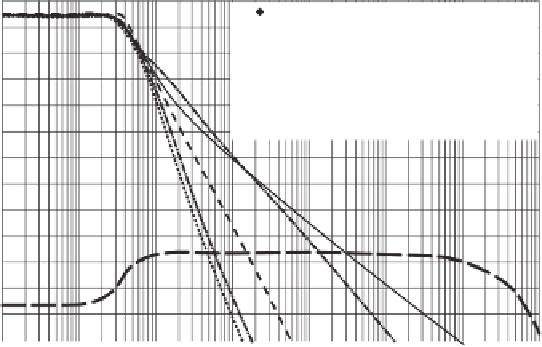










Search WWH ::

Custom Search