Biomedical Engineering Reference
In-Depth Information
-
-
-
Δ
/
h
D
/
h
= 0.04 ps
-1
D
/
h
= 0.01 ps
-1
=
0.003 ps
-1
-0.05
0
0.05
-0.05
0
0.05
-0.05
0
0.05
w
[ps
-1
]
w
[ps
-1
]
w
[ps
-1
]
Fig. 9.17
The transition from the “different dots” regime to the “identical dots” regime as
manifested by the form of the absorption spectrum of a DQD system. Here,
01 ps
−
1
and the
Γ
=
0
.
values of
h
are shown in the figures.
Red lines
: the actual system;
blue lines
: the hypothetical
system made of two dots interacting with separate reservoirs [
124
]
Δ
/
2
2
For
Δ
<
h
Γ
/
2,
Ω
+
=
(
Δ
/
h
)
−
Γ
/
4 is imaginary and the absorption spec-
trum can be written as
)=
Γ
+
|
Ω
+
|
Γ
+
Γ
−
|
Ω
+
|
Γ
−
(
ω
|
α
=
,
=
,
Δ
<
Γ
/
+
−
−
,
Im
P
0
V
0
h
2
2
2
2
2
4
ω
+
Γ
4
ω
+
Γ
where
0,
and thus the width of the first Lorentzian becomes twice larger than in the case
of emission to separated reservoirs while the width of the second one vanishes.
Since the transition from a single exponential decay rate to the twice larger one is a
signature of the existence of a short-living state, the first Lorentzian corresponds to
the superradiant component of the evolution. On the other hand, the transition which
leads to quenching of the decay rate is a fingerprint of subradiant character of the
second (negative) component. As can be seen in Fig.
9.16
b and d, the amplitudes
of the two Lorentzians are always opposite and thus the condition
P
Γ
±
=
Γ
±
2
|
Ω
+
|
. In the limit of
Δ
→
0 the decay rates
Γ
+
→
2
Γ
and
Γ
−
→
0is
fulfilled. These spectral features are reflected in the time domain. In the intermediate
range of
(
ω
=
0
)=
2) the decay of polarization becomes a sum of two
exponential factors: one positive, large and short living and the other one, negative,
small and long living. As seen in Fig.
9.16
a and c, also in this range it is hard to
distinguish systems interacting with common reservoirs from the ones emitting to
disjoint energy ranges where the oscillations are strongly damped. The analysis
of the emitted signal in the time domain allows to specify the coupling only in
the case of nearly identical dots (
Δ
(
Δ
slightly below
h
Γ
/
2) where for the collectively coupled
systems the initial response is dominated by the superradiant component and thus
the polarization decays with a twice as large rate compared to the dots coupled to
separate reservoirs (red lines in Fig.
9.16
aandc).
In Fig.
9.17
, we compare the transition between the limiting cases of non-
identical and identical quantum dots for systems coupled to common and separate
reservoirs. As long as the fundamental transition energies of the two dots differ
considerably (
Δ
h
Γ
/
2) the absorption spectrum of both types of systems is
indistinguishable, since the dots emit into disjoint frequency ranges. The situation
Δ
h
Γ
/
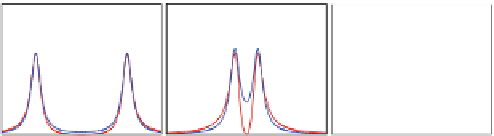
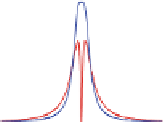
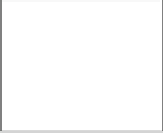






























































































