Biomedical Engineering Reference
In-Depth Information
(
Coriolus hirsitus
) cathode had [Os(4,4
-dimethyl-2,2
-bipyridine)
2
(2,2
,6
,2
-terpyri-
dine)]
2
conjugated to a similar polymer backbone, redox potential of
0.55 V vs Ag/
AgCl, as mediators. The cell was studied under conditions appropriate for high activity
of the laccase enzyme: aerated pH 5 citrate buffer and chloride-free medium containing
15 mM glucose. Maximum power density reached 137
Wcm
2
at 37ºC. The power
dropped by less than 10% after a day of continuous operation and by 25% after 3 days.
The cell power output was then refi ned [41] by the use of an anodic redox polymer
with a more appropriate redox potential of
µ
0.29 V and a fl exible tether to improve
charge transport diffusion. In this case an [Os(N,N
-biimidazole)
3
]
2
redox active moiety was tethered to the polymer backbone via one of its alkylated
biimidazole ligands through a 13 atom long fl exible spacer. At 37ºC the maximum
power density for the cell was 268
-alkylated-2,2
Wcm
2
at 0.78 V. Under continuous operation for
a week at 0.78 V and 37ºC the cell power dropped by 10% a day. The same refi nement
procedure was followed for the cathode [94], where an [Os(4,4
µ
-dimethyl-2,2
-bipyrid-
-bipyridine)]
2
complex was reacted with partially
quaternized polyvinylpyridine to yield an 8 atom long fl exible tether. The power den-
sity of this miniature biocatalytic fuel cell peaked (350
ine)
2
(4-aminomethyl-4
-methyl-2,2
Wcm
2
) at 0.88 V, the highest
cell voltage to date for a working miniature glucose-O
2
biocatalytic fuel cell, and just
300 mV below the thermodynamic potential of the glucose-O
2
cell reaction.
Along the principles just delineated above and almost concomitantly, the same
group developed their own GOx-BOD fuel cell, for reasons already discussed. The
refi nement of the biocatalytic fuel cell was conducted in pseudo-physiological con-
ditions of pH 7.4, 20 mM phosphate buffer containing 0.15 M NaCl and 15 mM glu-
cose, air saturated solution with oxygen concentration
µ
0.2 mM at 37ºC. In all cases
the cathode redox polymer consisted of a polyvinylimidazole-polyacrylamide co-
polymer functionalized with [Os(4,4
-bipyridine)
2
Cl]
, redox potential
-dichloro-2,2
of
0.35 V vs Ag/AgCl. The anode redox polymer was initially [Os(4,4
-diamino-2,2
-
bipyridine)
2
Cl]
, redox potential of
0.16 V vs Ag/AgCl, complexed to a partially
quaternized polyvinylimidazole [81]. The assembled biocatalytic fuel cell power den-
sity was 50
Wcm
2
at 0.5 V. Two days of continuous operation resulted in 40% loss
of the initial power density. Using a redox polymer containing [Os(4,4
µ
-dimethoxy-
2,2
0.07 V vs Ag/AgCl, for
the anode [95], the maximum power density increased signifi cantly to 244
-bipyridine)
2
Cl], with a slightly more anodic potential of
Wcm
2
at the lower voltage of 0.36 V. This illustrates the limiting effect of the anode on the
biocatalytic fuel cell performance under these conditions. One day of continuous
operation of this cell resulted in less than 10% loss at 0.36 V, and 45% loss after 4
days. Further refi nement of the anode involved, as was the case for the GOx-laccase
fuel cell, introduction of a long fl exible spacer between the polymer and [Os(N,N
µ
-
-biimidazole)
3
]
2
/3
redox active moiety to improve diffusional charge
transport between the enzyme active site and the electrode [96]. Despite the lower-
ing of the redox potential to
alkylated-2,2
0.19 V vs Ag/AgCl, the cell produced a power den-
Wcm
2
at the relatively high voltage of 0.52 V. One week of continuous
operation at 0.52 V resulted in an
sity of 430
µ
6% loss in power density per day. The operation
of this cell in a living plant has been demonstrated [97]. A grape sap was chosen for
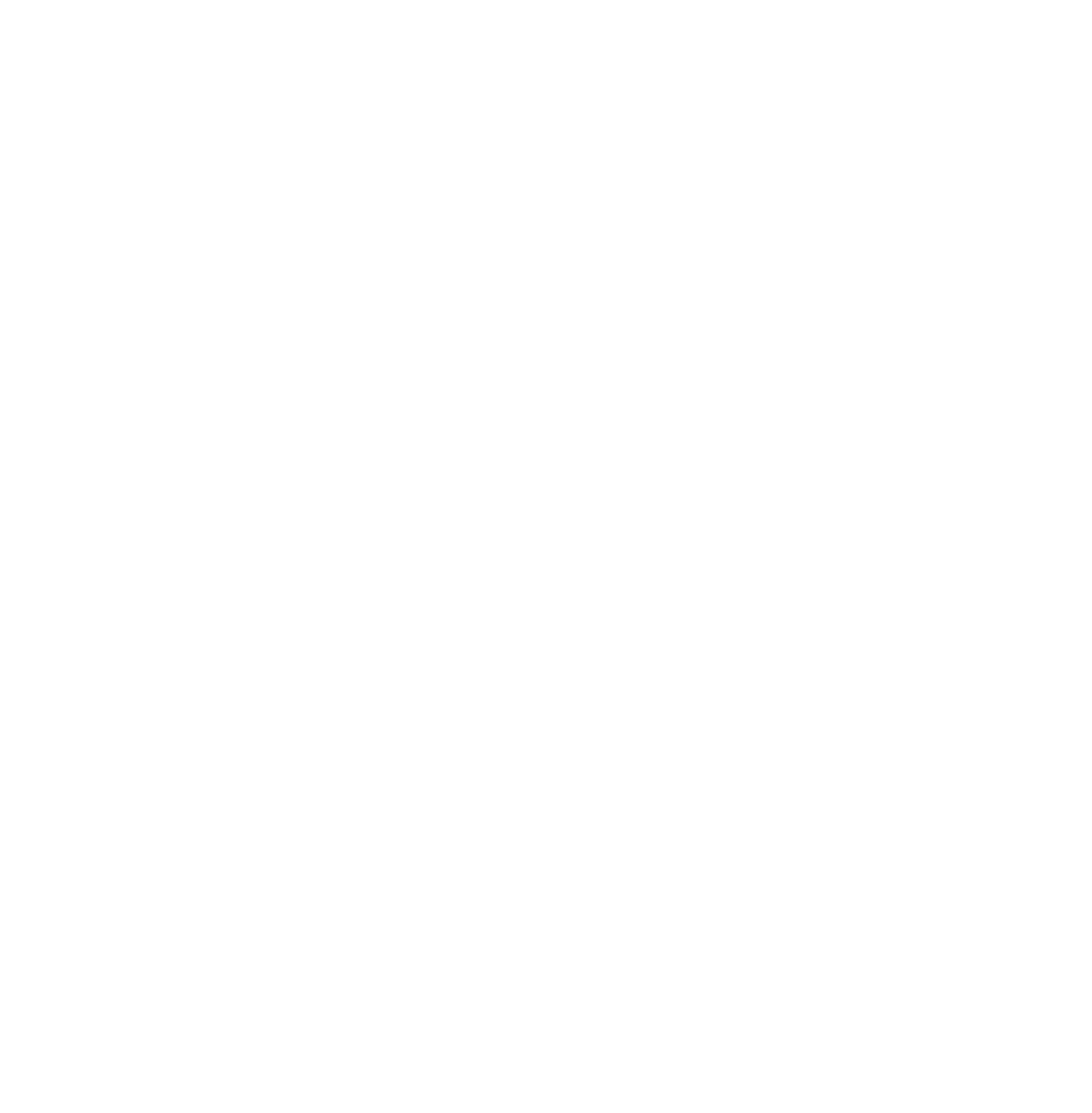




Search WWH ::

Custom Search