Biomedical Engineering Reference
In-Depth Information
constructed an immunosensor by binding the antigen
-fetoprotein to a polyaniline-anti-
body backbone on an electrode [63]. Nyquist plots were obtained sequentially in the pres-
ence of [Fe(CN)
6
]
3
/4
at the bare electrode, followed by depositing polyaniline fi lm,
immobilizing antibody and binding antigen to the antibody. A semicircle feature was
obtained when a bare electrode was used, indicating electron transfer kinetics at the elec-
trode. The diameter of the semicircle increased after each of the remaining three steps in
constructing the immunosensor. This was attributed to an increase in the charge transfer
resistance for [Fe(CN)
6
]
3
/4
after each layer was applied sequentially to the electrode
surface. Based on a calibration plot of
R
ct
vs
α
α
-fetoprotein concentration, a linear range
from 200 to 800 ng mL
1
of
-fetoprotein was obtained. Similarly, Yang
et al.
exam-
ined the impedance data obtained in the presence of [Fe(CN)
6
]
3
/4
after immobilizing
an immunocomplex involving
E. coli
at an IDA microelectrode [64]. The results showed
that immobilizing antibodies and binding
E. coli
cells to the IDA microelectrode surface
increased the electron transfer resistance, which was correlated with the concentration of
E. coli
from 4.36
α
10
8
cfu mL
1
with a detection limit of 10
6
cfu mL
1
.
In this section, we will make a brief mention of conductimetric immunosensors, which
are essentially based on the same principles as impedimetric immunosensors, except that
the change in conductance is measured as a result of antibody-antigen interaction rather
than impedance. Therefore, a label-free conductimetric immunosensor has also been
developed to detect the interaction between analyte rabbit IgG and immobilized anti-
rabbit goat IgG [68]. The goat antibody was physically trapped in a layer of 3,4-ethylen-
edioxythiophene on gold coated polycarbonate membranes. Detection was based upon the
change in conductance due to a change in the conformation of the conducting polymer
before and after the interaction between the immobilized and analyte IgGs.
10
5
to 4.36
5.6 MICROFLUIDIC ELECTROCHEMICAL IMMUNOASSAY
SYSTEMS
There have been vigorous efforts made to develop miniaturized fl ow systems in several
different areas. Progress made in adapting microfl uidic technology to immunoassays
has recently been reviewed by Bange
et al.
[7]. In this respect, microbead-based elec-
trochemical immunoassay is particularly suited to the development of microfl uidic
devices. Such work usually involves binding biotinylated antibody to streptavidin
coated microbeads of low-micron diameter to build the necessary immunocomplex.
The beads are polystyrene with iron particles dispersed throughout, giving rise to
their paramagnetic behavior. Owing to the small size of the beads, they are extremely
mobile and easily introduced into the channels of a microfl uidic device and their
movement can be easily controlled by a magnetic fi eld [2]. Moreover, dispersion of the
beads throughout a solution shortens both reagent diffusion distances and assay times.
There are continuing goals in this area to minimize the amounts of necessary reagents
used and the waste produced, while improving the detection limits [69]. In microbead-
based immunoassays involving enzyme labels, a direct consequence of the small
volumes used is that dilution of the enzyme product can be avoided, which aids in

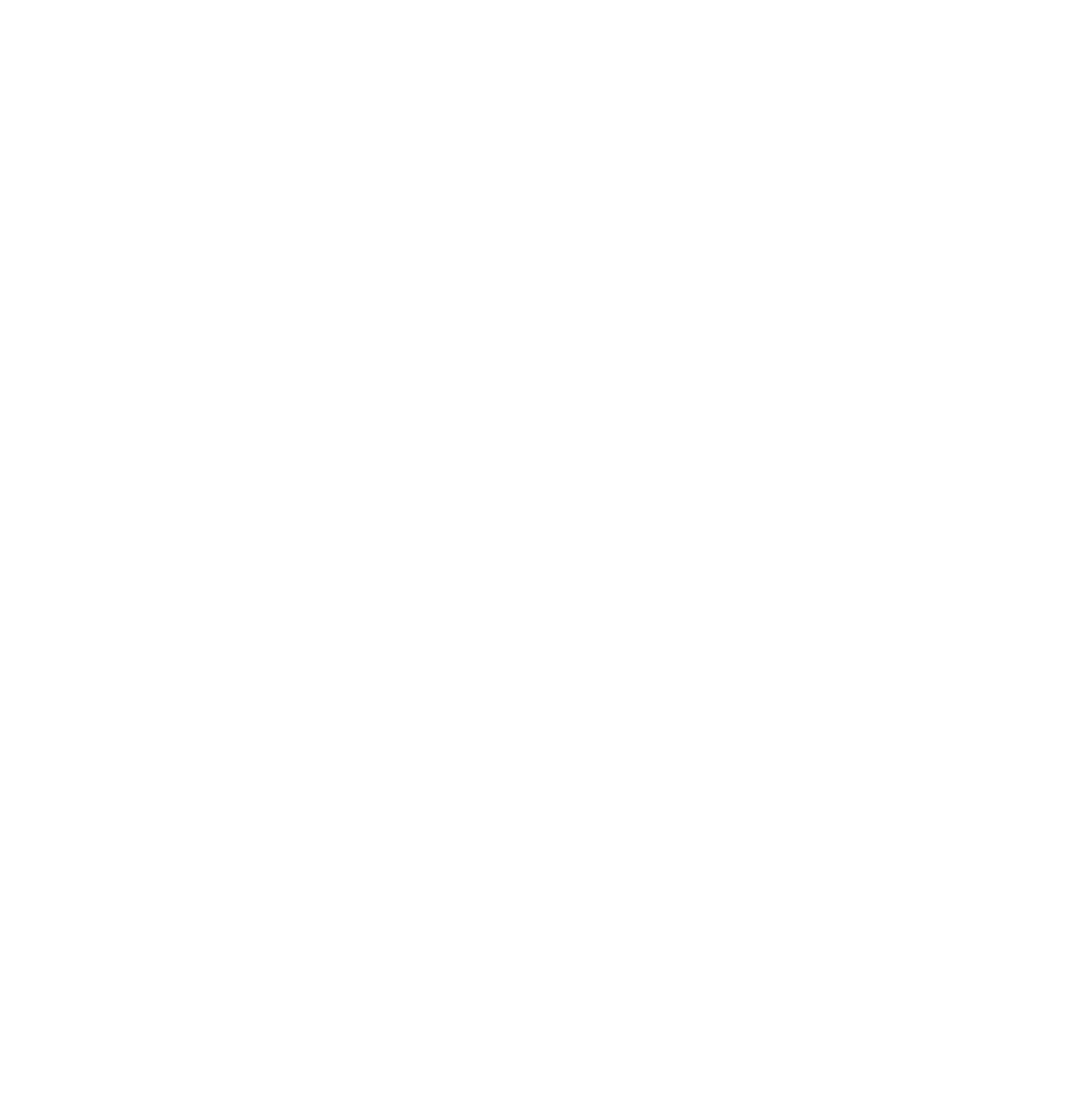




Search WWH ::

Custom Search