Biomedical Engineering Reference
In-Depth Information
ionophores may be provisionally divided into two main groups, as macrocyclic and
acyclic compounds. The fi rst, larger, group includes crowns, cryptands, calixarenes,
and cyclic polypeptides, which due to different and variable cavity size may spatially
discriminate ions. The high selectivity of macrocyclic ionophores is ensured by the
cavity size, but also by high preorganization of ionophore molecules, when its original
ion-free structure resembles one after complexation. Under such conditions the confor-
mational change during complex formation is usually of low energy. In preorganized
cavities cations may simultaneously interact with several functionalities, which is ener-
getically favorable. The cavity may be ring shaped in crowns, barrel or cylinder shaped
in calixarenes and hemispherical in bridged crown ionophores. The best-fi t approach,
based on cavity size and ionic radius, however, is not the only option, as crowns may
form complexes with a 1:2 ion-ionophore stoichiometry, which was used for suc-
cessful development of bis-crown ionophores, forming intramolecular “sandwiches”,
where an ion is squeezed between two crowns [76]. Many macrocyclic compounds and
especially calixarenes could be easily functionalized with additional chelating moie-
ties to enhance their selectivity and with branched alkyl chains to increase ionophore
lipophilicity and to avoid crystallization in the membrane [77].
Acyclic ionophores, although sometimes considered less selective than their mac-
rocyclic counterparts, are often of practical relevance. Open-chained analogs of crown
compounds and cryptands, so-called podands, are widely spread. Besides, amide deriv-
atives such as the well-known calcium ionophores ETH 1001 and ETH 129 (Fig. 4.1)
exhibit extremely high selectivities and are successfully used for calcium determina-
tion in physiological samples [78]. The complexes formed by such ionophores are
often of high stoichiometry, so usually two or three ionophore molecules enlace one
ion. Stabilization of the resulting complex is achieved due to a chelate effect. Rational
design of tweezer type ionophores [79], based on a rigid structure of cholic acid
derivatives (Fig. 4.1, CO
2
1) is another successful approach to acyclic ionophores.
Modifi cation at certain and variable positions of cholic acid with functionalities of dif-
ferent types gives a preorganized ionophore that holds the binding sites, spatially ori-
ented as tweezers. Depending on the binding site nature, ionophores may specifi cally
interact with either cations or anions, allowing signifi cant selectivity improvement.
Amid the prosperity of cation-carrier development, advances in anion-selective
ionophores so far seem to be modest. Larger size and diversity of anion geometries,
higher hydration and less solvation energies for anions than for cations of comparable
size, presence of different forms of anions in solution - all these factors hinder the
development of anion sensors [80]. The general principles of host-guest and supramo-
lecular chemistries are widely applicable for anion receptor design, but with certain
singularities [81, 82]. The development of modern anion ionophores usually exploits
two main processes: coordination to a metal center and hydrogen bonding with ion-
dipole interactions. The best results are often obtained by combination of these two
features in a single receptor.
Organometallic ionophores containing either metal ion complexed with lipophilic
organic ligands, or a metal atom covalently bound to organic molecules may serve
as charged or neural carriers. Probably the most interesting class of organometallic
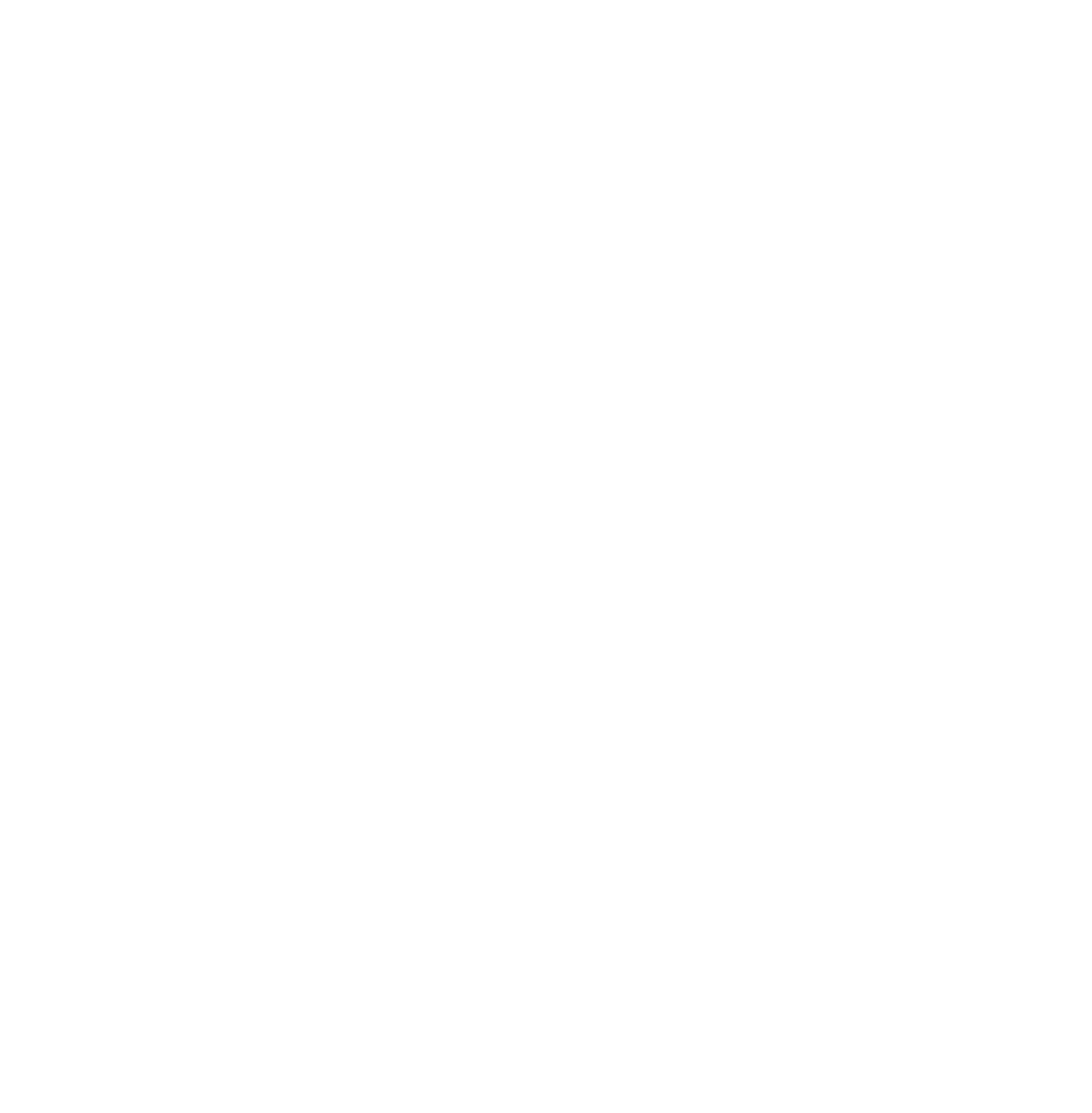




Search WWH ::

Custom Search