Biomedical Engineering Reference
In-Depth Information
Figure 4.
Non-wetting (
θ>
90
◦
).
one. These angles fall within a certain range with advanced angles approaching
the maximum value ('advancing') and receded angles approaching minimum value
('receding'). The difference between advancing and receding contact angle is called
the contact angle hysteresis. Hysteresis phenomena are attributed to surface rough-
ness, mobility and chemical heterogeneity [5]. For inhomogeneous surfaces, any de-
fect will present barriers to the motion of the three-phase contact line and metastable
states may occur [6, 7]. Hysteresis effect can also be viewed as an indication of the
extent of the change in surface properties caused by wetting [8]. Lam
et al
.have
shown that hysteresis depends on the size of liquid molecules and that both advanc-
ing and receding contact angles decrease with liquid sorption/retention [9]. Other
works investigated the influence of drop size [10] or coverage and distribution of
the surfactants [11] on contact angle hysteresis.
Another important parameter for illustration of wetting behaviour is the spread-
ing coefficient,
S
. The spreading coefficient is defined as the difference in free
energies between the bare solid directly in contact with the gaseous phase and the
solid covered by a flat liquid layer [1].
S
γ
lg
),
(2)
γ
sg
here represents the interfacial tension of the 'dry' surface. For
S<
0, the liquid
will form a drop with finite contact angle (partial wetting). For
S
=
γ
sg
−
(γ
sl
+
0 drop will
flatten forming a thin film spread over the surface (complete wetting with
θ
eq
=
0).
C. Spreading of Pure Liquids
When a liquid drop is placed onto a flat surface, capillary forces drive the interface
spontaneously towards equilibrium. At the same time, there is hydrodynamic resis-
tance to spreading that opposes the capillary driving force. Evolution of the drop
radius and the contact angle with time are commonly investigated to describe the
dynamics of wetting on solid surfaces. For pure liquids, three theoretical approaches
have been developed to describe spreading of the drop: the hydrodynamic model
[11-14], the molecular-kinetic model [15-17] and the evaporation/condensation
model [18-20]. The main difference between the former two descriptions is the
dominant energy dissipation channel during drop shape transformation. The dis-
sipation of energy in the hydrodynamic model is due to viscous drag within the
spreading droplet, unlike the molecular-kinetic model where the dissipation of en-
ergy is assumed to be caused by friction at the three-phase contact line (intermolec-
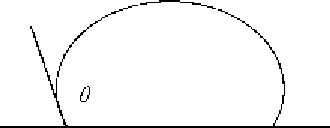
Search WWH ::

Custom Search