Biomedical Engineering Reference
In-Depth Information
Systems models provide better clarity, particularly
with regard to information flow or influences. In analog
models, all components may interact to some extent and
this interaction may not be obvious from inspection of
the model. Referring to the eye muscle model of
Figure 2.3-7
, a change in just one parallel elastic element,
k
P
,
, will modify the force on every element in the model.
In systems models, all influences are explicitly shown and
their interactions are immediately apparent from an in-
spection of the model. For example, in the model of
Figure 2.3-9
, the fact that the iris also influences the
neural controller is explicitly shown by the feedback
pathway. This can be of great benefit in clarifying the
control structure of a complex system. Perhaps the most
significant advantage of the systems approach is that it
allows processes to be rigorously represented without
requiring the modeler to know the details of the un-
derlying physiological mechanism.
such as filtering are not usually successful. Sometimes
environmental noise can be reduced using
adaptive filters
or
noise cancellation
techniques that adjust their filtering
properties on the basis of the current environment.
Measurement artifact is produced when the mea-
surement device, or
transducer,
responds to energy mo-
dalities other than those desired. For example, recordings
of electrical signals of the heart, the ECG, are made using
electrodes placed on the skin. These electrodes are also
sensitive to movement, so-called
motion artifact,
where
the electrodes respond to mechanical movement as well
as to the desired electrical activity of the heart. This ar-
tifact is not usually a problem when the ECG is recorded
in the physician's office, but it can be if the recording is
made during a patient's normal daily living, as in a Holter
recording. Measurement artifacts can sometimes be suc-
cessfully addressed by modifications in transducer design.
Aerospace research has led to the development of elec-
trodes that are relatively insensitive to motion artifact.
Unlike the other sources of variability, electronic noise
has well-known sources and characteristics. Electronic
noise falls into two broad classes:
thermal
or
Johnson
noise, and
shot
noise. The former is produced primarily in
resistor or resistance materials whereas the latter is re-
lated to voltage barriers associated with semiconductors.
Both sources produce noise that contains energy over
a broad range of frequencies, often extending from DC to
10
12
to 10
13
Hz. Such broad-spectrum noise is referred
to as
white noise
because it contains energy at all fre-
quencies (or at least all the frequencies of interest to
bioengineers) just as white light contains energy at all
frequencies (or at least, all the frequencies we can see).
Figure 2.3-11
shows a plot of the energy in a simulated
white-noise waveform (actually, an array of random
numbers) plotted against frequency. This is similar to
a plot of the energy in a beam of light versus wavelength
(or frequency) and, as with light, is also referred to as
a
spectral plot
or
spectrum.
Note that the energy of the
simulated noise is constant across the spectral range.
The various sources of noise or variability along with
their causes and possible remedies are presented in
Table 2.3-3
. Note that in three of four instances, appro-
priate transducer design may aid in the reduction of the
variability or noise. This demonstrates the important role
of the transducer in the overall performance of the
instrumentation system.
2.3.4 Noise and variability
Where there is signal, there is noise. Occasionally, the
noise will be at such a low level that it is of little con-
cern, but usually the noise limits the usefulness of the
signal. This is especially true for physiological signals
because they experience many potential sources of noise
or variability. In most usages,
noise
is a general and rel-
ative term: noise is what you do not want, and signal is
what you do want. This leads to a definition of noise as
any form of unwanted variability. Noise is inherent in
most measurement systems and is often the limiting factor
in the performance of a medical instrument. Indeed, many
medical instruments go to great lengths in terms of signal-
conditioning circuitry and signal-processing algorithms to
compensate for unwanted variability.
In biomedical measurements, noise or variability has
four possible origins: (a) physiological variability;
(b) environmental noise or interference; (c) measurement
or transducer artifact; and (d) electronic noise. Physio-
logical variability comes about because the information
you desire is based on measurements subject to biological
influences other than those of interest. For example,
assessment of respiratory function based on the mea-
surement of blood Po
2
could be confounded by other
physiological mechanisms that alter blood Po
2
. Measure-
ment errors due to physiological variability can be very
difficult to resolve, sometimes requiring a total redesign
(or rethinking) of the approach.
Environmental noise can come from sources external
or internal to the body. A classic example is the mea-
surement of the fetal ECG signal where the desired
signal is corrupted by the mother's ECG. Because it is
not possible to describe the specific characteristics of
environmental noise, typical noise reduction approaches
2.3.4.1 Electronic noise
Johnson or thermal noise is produced by resistance
sources and the amount of noise generated is related to
the resistance and to the temperature:
V
J
ΒΌ
p
4
k TRBW
volts
[Eq. 2.3.8]

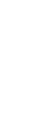




