Biomedical Engineering Reference
In-Depth Information
applied magnetization and dephases. Time
T
1
governs the
rate of recovery of the longitudinal magnetization.
In summary, the spin-spin relaxation time
T
2
is the
time to reduce the transverse magnetization by a factor
of
e
. In the previous sequence,
T
2
and
T
1
processes are
shown separately for clarity. That is, the magnetization
vectors are shown filling the
XY
plane completely before
growing back up along the
Z
axis. Actually, both processes
occur simultaneously with the only restriction being that
T
2
is less than or equal to
T
1
.
Two factors contribute to the decay of transverse
magnetization:
1.
molecular interactions (said to lead to a pure
T
2
molecular effect)
2.
variations in
B
0
(said to lead to an inhomogeneous
T
2
effect)
The combination of these two factors is what actually
results in the decay of transverse magnetization. The
combined time constant is called
T
2
:
The relationship
between the T
2
from molecular processes and that from
inhomogeneities in the magnetic field is as follows.
absorbing a photon with energy matching the energy
difference between the two states. This means that when
nuclei under the effect of an applied magnetic field are
irradiated by photons in the form of electromagnetic
fields at a certain frequency by an RF probe, some nuclei
in the lower energy states will absorb the photons and
jump to a higher energy state. After the radiated energy is
interrupted, the nuclei in the higher energy state will
return to the lower energy state to recover to equilib-
rium, emitting photons on electromagnetic fields, which
can be detected by an MRI probe. The frequency emitted
by the electromagnetic fields is detected by the energy
difference between the two states of the nuclei. The
decay of the signals in time depends on the molecular
environment of the nuclei. Because the energy difference
between the two states of a given nucleus in an external
field depends on the strength of the external field, the
energy difference at any point in the body can be made
different by varying the magnetic field strength from
point to point. Therefore, the energy of the photons and
the frequency of the electromagnetic fields absorbed and
emitted by the nuclei are also different from point to
point. When the signals from all nuclei are received, their
frequency can be used to determine spatial information
about the nuclei.
From quantum mechanics certain atomic nuclei pos-
sess what is called spin. A proton, which has a mass, can
be thought of as spinning, as shown in
Figure 6.1-7
with
its own angular momentum. The circulating positive
current creates a small magnetic field.
Neutrons can also be thought of as generating a small
magnetic field since their distributed positive and nega-
tive charges are not uniformly distributed. These small
magnetic fields are called magnetic moments
M
and are
given by
1
T
2
¼
1
T
2
1
T
2 inhomo
þ
(6.1.8)
MRI is based on the physical principle of NMR. NMR
was discovered by Black and Purcell in 1946. When
certain atomic nuclei are placed in a static magnetic field,
it will assume either a higher energy level or a lower
energy level (
Figure 6.1-6
). As shown in the figure, the
energy between the two states is linearly dependent on
the strength of the applied static field: a physical prin-
ciple known as the Zeeman effect. A nucleus in a higher
energy state can fall to the lower energy state. A nucleus
will emit a photon when transitioning between these two
energy states. The energy of the photon is equal to the
energy difference between the two states. A nucleus in
the lower energy state can jump to a higher energy state,
M ¼
g
J
(6.1.9)
M
High Energy State
e
e
Nuclei
energy
+
+
+
+
En
+
+
+
+
e
Low Energy State
Magnetic Field Strength
Figure 6.1-6 Energy level of nuclei under a magnetic field.
Figure 6.1-7 Magnetic field precession in magnetic fields.


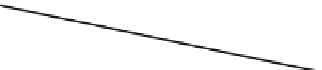





























