Biomedical Engineering Reference
In-Depth Information
Table 3.2.16-4 Examples of immobilized enzymes in therapeutic
bioreactors
Table 3.2.16-5 Some advantages and disadvantages of
immobilized enzymes
Advantages
Enhanced stability
Can modify enzyme microenvironment
Can separate and reuse enzyme
Enzyme-free product
Lower cost, higher purity product
No immunogenic response (therapeutics)
Medical
application
Substrate
Substrate
action
Cancer treatment
L
-Asparaginase
Asparagine
Cancer cell
nutrient
L
-Glutaminase
Glutamine
Cancer cell
nutrient
Disadvantages
Difficult to sterilize
Fouling by other biomolecules
Mass transfer resistances (substrate in and product out)
Adverse biological responses of enzyme support surfaces (in vivo
or ex vivo)
Greater potential for product inhibition
L
-Arginase
Arginine
Cancer cell
nutrient
L
-Phenylalanine
lyase
Phenylalanine
Toxin
Indole-3-alkane
a
hydroxylase
Tryptophan
Cancer cell
nutrient
Cytosine
deaminase
5-Fluorocytosine
Toxin
Liver failure (detoxification)
Bilirubin oxidase
lysines, whose
3
-amino groups may interact with pre-
adsorbed tissue plasminogen activator (tPA) during co-
agulation, to enhance fibrin clot dissolution at that surface.
Some of the advantages and disadvantages of immo-
bilized biomolecules are listed in
Table 3.2.16-5
, using
enzymes as an example.
Bilirubin
Toxin
UDP-gluceronyl
transferase
Phenolics
Toxin
Other
Heparinase
Heparin
Anticoagulant
Urease
Urea
Toxin
Immobilization methods
proteins. An approach using biologically functional ma-
terials can be much more direct, by adsorbing or co-
valently grafting ligands for cell-surface adhesion
receptors to the material surface. This has been accom-
plished with peptides grafted randomly over a substrate
(
Massia and Hubbell, 1991
) as well as with peptides
presented in a pre-clustered manner (
Irvine
et al.,
2001
).
The latter has important advantages: Cells normally
cluster their adhesion receptors into assemblies referred
to as focal contacts, and preassembly confers benefits in
terms of both adhesion strength (
Wa rd a nd H am mer,
1993
) and cell signaling (
Maheshwari
et al,
2000
). In
addition to peptides, saccharides have also been grafted
to polymer surfaces to confer biological functionality
(
Griffith and Lopina, 1998; Chang and Hammer, 2000
).
Specific biomolecules can be immobilized in order to
control cellular interactions; one important example is the
polypeptide growth factor. Such molecules can be
immobilized and retain their ability to provide biological
cues that signal specific cellular behavior, such as support
of liver-specific function in hepatocytes (
Kuhl and
Griffith-Cima, 1996
), induction of neurite extension in
neurons (
Sakiyama-Elbert
et al.,
2001
), induction of an-
giogenesis (
Zisch
et al.,
2001
), or the differentiation of
mesenchymal stem cells into bone-forming osteoblasts
(
Lutolf
et al.,
2003b
). Other molecules may be immobi-
lized that can partake in enzymatic reactions at the sur-
face.
McClung
et al.
(2001, 2003)
have immobilized
There are three major methods for immobilizing bio-
molecules (
Table 3.2.16-6
)(
Stark, 1971; Zaborsky, 1973;
Dunlap, 1974
). It can be seen that two of them are
physically based, while the third is based on covalent or
''chemical'' attachment to the support molecules. Thus, it
is important to note that the term ''immobilization'' can
refer either to a transient or to a long-term localization of
the biomolecule on or within a support. In the case of
a drug delivery system, the immobilized drug is supposed
to be released from the support, while an immobilized
Table 3.2.16-6 Biomolecule immobilization methods
Physical adsorption
van der Waals
Electrostatic
Affinity
Adsorbed and cross-linked
Physical ''entrapment''
Barrier systems
Hydrogels
Dispersed (matrix) systems
Covalent attachment
Soluble polymer conjugates
Solid surfaces
Hydrogels
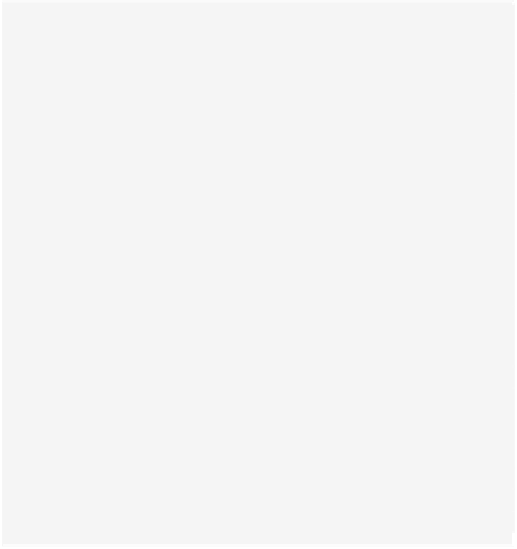



















