Biomedical Engineering Reference
In-Depth Information
degree on readily controllable factors such as the pres-
ence of plasticizers or additives, the manufacturing pro-
cess, the initial molecular weight of the polymer, and the
geometry of the device.
Unfortunately, the use of the highly dangerous ethylene
oxide gas represents a serious safety hazard as well as
potentially leaving residual traces in the polymeric
device. Polymers sterilized with ethylene oxide must be
degassed for extended periods of time.
Additionally, for applications in tissue engineering,
biodegradable scaffolds may be preseeded with viable
cells or may be impregnated with growth factors or other
biologics. There is currently no method that could be
used to sterilize scaffolds that contain viable cells with-
out damaging the cells. Therefore, such products must be
manufactured under sterile conditions and must be used
within a very short time after manufacture. Currently,
a small number of products containing preseeded, living
cells are in clinical use. These products are extremely
expensive, are shipped in special containers, and have
little or no shelf life.
Likewise, it has been shown that sterilization of scaf-
folds containing osteoinductive or chondroinductive
agents leads to significant losses in bioactivity, depending
on the sterilization method used (Athanasiou
et al.,
1998).
The challenge of producing tissue engineering scaffolds
that are preseeded with viable cells or that contain sen-
sitive biological agents has not yet been fully solved.
After sterilization, degradable implants are usually
packaged in air-tight aluminum-backed plastic-foil
pouches. In some cases, refrigeration may also be re-
quired to prevent backbone degradation during storage.
Storage stability, sterilization,
and packaging
It is important to minimize premature polymer degra-
dation during fabrication and storage. Traces of moisture
can seriously degrade even relatively stable polymers
such as poly (bisphenol A carbonate) during injection
molding or extrusion. Degradable polymers are particu-
larly sensitive to hydrolytic degradation during high-
temperature processing. The industrial production of
degradable implants therefore often requires the con-
struction of ''controlled atmosphere'' facilities where the
moisture content of the polymer and the ambient hu-
midity can be strictly controlled.
After fabrication, g -irradiation or exposure to ethyl-
ene oxide may be used for the sterilization of degradable
implants. Both methods have disadvantages and as
a general rule, the choice is between the lesser of two
evils.
g
-Irradiation at a dose of 2 to 3 Mrad can result in
significant backbone degradation. Since the aliphatic
polyesters PLA, PGA, and PDS are particularly sensitive
to radiation damage, these materials are usually sterilized
by exposure to ethylene oxide and not by
g
-irradiation.
Bibliography
Agrawal, C. M., Hass, K. F., Leopold, D. A.,
and Clark, H. G. (1992). Evaluation of
poly(
L
-lactic acid) as a material for
intravascular polymeric stents.
Biomaterials
13: 176-182.
Allcock, H. R. (1990). In
Biodegradable
Polymers as Drug Delivery Systems,
M.
Chasin, and R. Langer, eds. Marcel
Dekker, New York, pp. 163-193.
Anderson, J. M., Spilizewski, K. L.,and
Hiltner, A. (1985). In
Biocom-patibility
of Tissue Analogs,
Vol. 1, D. F. Williams,
ed. CRC Press Inc., Boca Raton, FL,
pp. 67-88.
Andrianov, A. K., Sargent, J. R., Sule, S. S.,
LeGolvan, M. P., Woods, A. L., Jenkins,
S. A., and Payne, L. G. (1998).
Synthesis, physico-chemical properties
and immunoadjuvant activity of water-
soluble polyphosphazene polyacids.
J. Bioactive Comp. Polym.
13: 243-256.
Athanasiou, K., Agrawal, M., Barber, A.,
and Burkhart, S. (1998). Orthopaedic
applications for PLA-PGA
biodegradable polymers.
Arthroscopy
14: 726-737.
Attawia, M. A., Uhrich, K. E., Botchwey, E.
, Fan, M., Langer, R., and Laurencin,
C. T. (1995). Cytotoxicity testing of
poly(anhydride-co-imides) for
orthopedic applications.
J. Biomed.
Mater. Res.
29: 1233-1240.
Barham, P. J., Keller, A., Otun, E. L., and
Holmes, P. A. (1984). Crystallization
and morphology of a bacterial
thermoplastic: poly-3-hydroxybutyrate.
J. Mater. Sci.
19: 2781-2794.
Beele, H. (2002). Artificial skin: Past,
present and future.
Int. J. Artificial
Organs
25: 163-173.
Bergsma, J. E., de Bruijn, W. C., Rozema,
F. R., Bos, R. R. M., and Boering, G.
(1995). Late degradation tissue
response to poly(l-lactic) bone plates
and screws.
Biomaterials
16: 25-31.
Campbell, P., Glover, G. I., and Gunn,
J. M. (1980). Inhibition of intracellular
protein degradation by pepstatin,
poly(
L
-lysine) and pepstatinyl-poly
(
L
-lysine).
Arch. Biochem. Biophys.
203:
676-680.
Chasin, M., Domb, A., Ron, E.,
Mathiowitz, E., Langer, R., Leong, K.,
Laurencin, C., Brem, H., and
Grossman, S. (1990). In
Biodegradable
Polymers as Drug Delivery Systems,
M. Chasin and R. Langer, eds. Marcel
Dekker, New York, pp. 43-70.
Choueka, J., Charvet, J. L., Koval, K. J.,
Alexander, H., James, K. S., Hooper,
K. A., and Kohn, J. (1996). Canine bone
response to tyrosine-derived
polycarbonates and poly(
L
-lactic acid).
J. Biomed. Mater. Res.
31: 35-41.
Deshpande, A. A., Heller, J., and Gurny, R.
(1998). Bioerodible polymers for ocular
drug delivery.
Crit. Rev. Thera. Drug
Carrier Syst.
15: 381-420.
Frazza, E. J., and Schmitt, E. E. (1971).
A new absorbable suture.
J. Biomed.
Mater. Res.
1: 43-58.
Gilding, D. K., and Reed, A. M. (1979).
Biodegradable polymers for use in
surgerydpoly(glycolic)/poly(lactic
acid) homo- and copolymers: 1.
Polymer
20: 1459-1464.

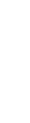




