Geology Reference
In-Depth Information
been widely verified. However, the approach is promising
(pending on validation) because it can be used to generate
albedo maps over the polar region at least weekly (the
entire Arctic is covered by the wide swath mode of space-
borne SAR in 3‐4 days) regardless of cloud masking or
atmospheric influences.
Spectral albedo is affected by changes in the physical
and polycrystalline properties of sea ice and snow. In
principle, high albedo values result from physical changes
that enhance scattering. This includes the formation of
air bubbles in MY ice or air voids in fresh snow cover
[
Perovich
, 1996]. On the other hand, low albedo values
result from conditions that enhance the absorption. This
includes the presence of liquid water in the snow volume
or at the bare ice surface. The decrease of albedo due to
the presence of liquid water in the snow volume is more
pronounced at longer wavelengths. Another noteworthy
remark relates albedo to brine pocket composition in sea
ice. As discussed in section 2.3, brine pockets within the
polycrystalline sea ice matrix expand or shrink as ice tem-
perature decreases or increases, respectively. Upon expan-
sion, more absorption of optical radiation takes place
and vice versa. Below the eutectic temperature of the
sodium chloride (−22.8 °C) this salt precipitates in brine
pockets. This turns the pockets into scattering elements
of the radiation instead of being absorbents. As a result,
the albedo of sea ice below this temperature is very high
(close to 1) as reported in
Grenfell
[1983]. This tempera-
ture is common in the upper layers of polar sea ice in
winter and early spring.
A final note is worth included about spectral albedo
from sediment‐laden sea ice. A few studies have demon-
strated that a substantial fraction of the ice surface in the
Arctic is covered with sediments in the summer, which
results in significant reductions in albedo [
Light et al
.,
1998;
Tucker et al.,
1999]. Sediments are usually entrained
during ice formation over the shallow Arctic shelves and
often concentrate into layers of mud several millimeters
thick [
Nürnberg et al
., 1994].
Arrigo et al.
[1991] modeled
the effect of biogenic materials such as algae on the
albedo of Antarctic sea ice.
Huck et al.
[2007] computed
the spectral albedo for different ice surfaces of variable
sediment loads with a radiative transfer model for sea ice
coupled with an optical model for particulates included
in sea ice. This was necessary in order to utilize satellite
observations for mapping the extent of particle‐laden sea
AVHRR Ch.2
AVHRR Ch.1
1. 0
Clean ice
Clean ice
25 g/m
3
50 g/m
3
0.8
75 g/m
3
200 g/m
3
400 g/m
3
500 g/m
3
1000 g/m
3
0.6
Light pond
Light pond
Dark pond
0.4
Dark pond 100 g/m
3
Dark pond 300 g/m
3
Open water
0.2
Dark pond 100 g/m
3
Open water
0.0
400
600
800
1000
1200
1400
Wavelength (nm)
Figure 8.28
Modeled surface spectral albedo for sediment‐laden ice with bare surface and ponded ice underlain
by the same sediment‐laden ice. Data simulate the situation under direct incident illumination with solar zenith
angle 50°. Total sediment loading is indicated for each curve. For ponded ice (dark pond), total particulate load-
ings were concentrated within the uppermost 1 cm of the ice beneath the pond water. Spectral bands of AVHRR
channels 1 and 2 are indicated at the top of the graph [reproduced with modifications from
Huck et al.
, 2007,
Figure 2, with permission from Elsevier].
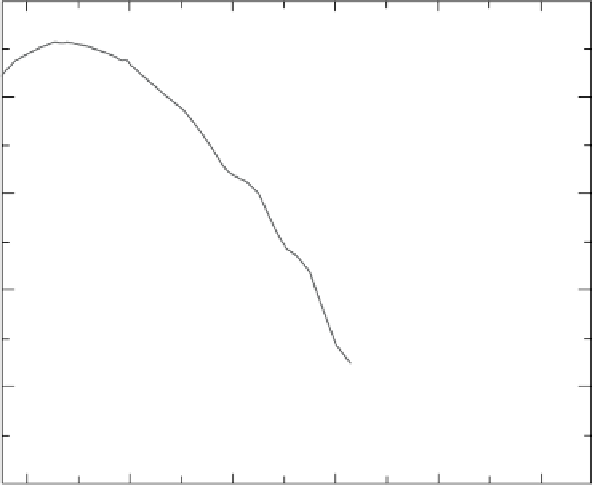
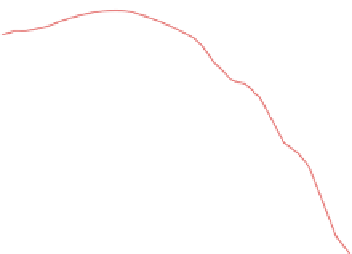
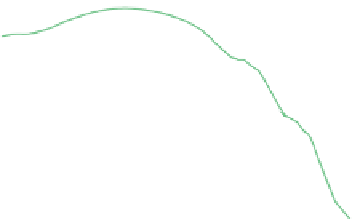


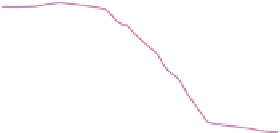
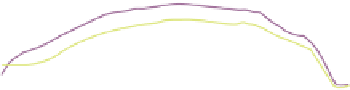
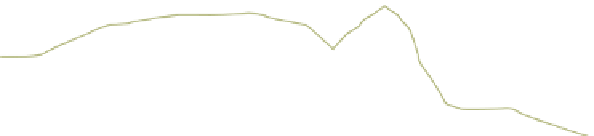


















