Biomedical Engineering Reference
In-Depth Information
channels between lamellar structures that could be widened by the introduction of
charged molecules into previously uncharged lecithin layers [18, 19]. These multila-
mellar liposomes were found to capture a variety of cationic species from tiny li
+
ions to relatively large cholines and, as soon to be shown, imaging reporters that were
dissolved in the aqueous phase at the time of liposome formation.
following the discovery and characterization of multilamellar liposomes,
D. Papahadjopoulos and N. Miller in 1967 described the structure of small unilamellar
vesicles (SUvs) [20, 21]. This was an important development, since SUvs could be
formed with better reproducibility and could serve as a technological platform for
molecular imaging.
1.4.2
visualization of liposomes
in Vivo
The majority of liposome clinical applications were historically centered in drug
delivery. However, the visualization of the liposome distribution
in vivo
was critical
for their clinical success and was the driving force behind the labeling of the
liposomes with imaging reporters. In the beginning of the 1970s, g. gregoriadis with
colleagues from the royal free Hospital School of Medicine in london prepared
liposomes labeled with entrapped
131
I-labeled albumin [22, 23] (fig. 1.6). Upon
in vivo
administration, these liposomes were primarily deposited into the liver (major)
HO
Cholesterol
131
I
H
2
C
OOCR'
O
3
H
R"COO
CH
P
CHCH
2
N(CH
3
)
3
H
2
C
O
O
Phosphatidylcholine
O
O
O
O
P
CH
2
CH
2
N(CH
3
)
3
O
O
O
O
H
O
1,2-Dihexadecanoyl-
sn
-glycero-3-
phosphocholine
figure 1.6
Design of
131
I-albumin liposomes. [
3
H]amyloglucosidase and
131
I-labeled
albumin were entrapped into liposomes composed of phosphatidyl choline, cholesterol, and
dicetyl phosphate.
131
I-labeled albumin was also entrapped in [
3
H]cholesterol liposomes.
(Based on refs. [22] and [23].)
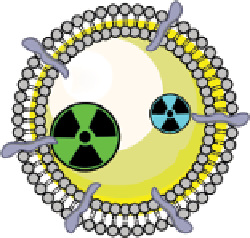
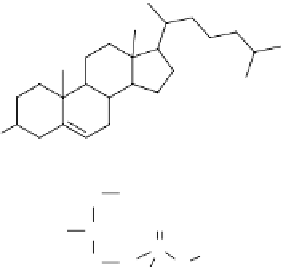


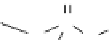




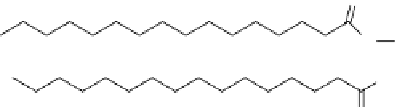






Search WWH ::

Custom Search