Environmental Engineering Reference
In-Depth Information
H
2
e
-
CB
2H
+
-1.0
-0.5
2H
+
+ 2e
-
H
2
2.0 eV
(Typical value)
h
υ
Cu
2
O + H
2
O + 2e
-
0.0
2Cu + 2OH
-
CuO generation
2CuO + H
2
O + 2e
-
Cu
2
O + OH
-
0.5
OH
-
h
+
VB
1.0
O
2
+ 2H
2
O + 4e
-
H
2
O
4OH
-
Cuprous oxide
(Cu
2
O)
figure 3.17
Fundamental principle of semiconductor-based photocatalytic water splitting for hydrogen generation. Reproduced by
permission from Ref. [199]. © 2008, The electrochemical Society.
oxidizing agent to produce H
2
and O
2
, respectively. Water splitting into H
2
and O
2
is an uphill reaction. It needs the standard Gibbs free
energy change Δ
G
0
of 237 kJ/mol, corresponding to 1.23 eV, as shown in equation 3.25:
HO OH
→+ =+
,
∆
G
237
kJ mol
/
(3.25)
2
2
2
Therefore, the band-gap energy (
E
g
) of the photocatalyst should be greater than 1.23 eV to achieve water splitting. Furthermore,
the width of the band gap and the potential of CB and VB are critical for efficient water splitting. The bottom level of the CB
has to be more negative than the reduction potential of H
+
/H
2
(0V versus NHe), while the top level of the VB has to be more
positive than the oxidation potential of O
2
/H
2
O (1.23 V).
Since the band-gap energy (
E
g
) of the photocatalyst should be greater than 1.23 eV to achieve water splitting, the band gap
of a semiconductor should be less than 3.0 eV (>400 nm) to use VL. However, TiO
2
is a wide band-gap semiconductor
(
E
g
= 3.2 eV), which limits the absorption of sunlight to the major portion of the solar spectrum. The approach to solving the
water-splitting problem has focused on exploring new materials for both anodic and cathodic processes and integrating config-
urations that utilize photovoltaic cell junctions. The ultimate goal of these efforts is an efficient photoelectrolysis cell design
that can simultaneously drive, in an unassisted fashion, both hydrogen evolution and water oxidation reactions. Water-splitting
cells require semiconductor materials that are able to support rapid charge transfer at a semiconductor/aqueous interface,
exhibit long-term stability, and efficiently harvest a large portion of the solar spectrum. In 1998, Hara et al. [30] reported that
illuminated Cu
2
O particles were needed for the evolution of H
2
and O
2
from water. Since then, Cu
2
O- and Cu
2
O-based semicon-
ductors were extensively investigated for photocatalytic water splitting. In Hara et al.'s experiments, H
2
and O
2
were evolved
over a period of 1900 h, without a noticeable decrease in activity. In later studies, they also observed that water splitting
continued under stirring conditions for many hours after the light was switched off [192]. This dark reaction was observed for
a variety of oxides including Cu
2
O, NiO, Co
3
O
4
, and Fe
3
O
4
with a reaction rate about one-third that of the photochemical exper-
iments with Cu
2
O. To explain their results, Ikeda et al. [193] proposed that the mechanical energy supplied by the stirring was
converted into chemical energy with the metal oxide acting as a catalyst. However, de Jongh et al. [194, 195] presented a neg-
ative result that the presence of Cu
2
O electrodes for reduction of water is kinetically unfavorable, although high photocurrent
efficiency was observed in the presence of oxygen or electron scavengers. Vacuum studies on the decomposition of d
2
O by
Cu
2
O using UV light, on the other hand, showed no evidence of photosplitting [196]. Most recently, photocatalysis by Cu
2
O
powders was investigated in distilled water, and it was found that hydrogen evolution was possible only with freshly prepared
Cu
2
O powder, while oxygen evolution could not be observed [197]. With a cathodic bias of −0.3V versus Ag/AgCl, Nian et al.
[198] directly evidenced that the photoinduced charges in the CB of p-type Cu
2
O are capable of reducing water to H
2
. Kakuta
and Abea [199] evaluated the photocatalytic potential of Cu
2
O powder by studying its ability to stoichiometrically decompose
water into O
2
and H
2
. Although thus far it has been indicated that Cu
2
O can function as a photocatalyst for overall water
splitting, H
2
evolution is found to take place solely when there is no generation of O
2
. Moreover, the evolution rate of H
2
grad-
ually decreases with prolonged irradiation, and, consequently, ceases. From the structural analyses of deactivated Cu
2
O, it
appears that H
2
evolution commences with the self-oxidation of Cu
2
O to CuO. From the band structure of Cu
2
O in Figure 3.17,
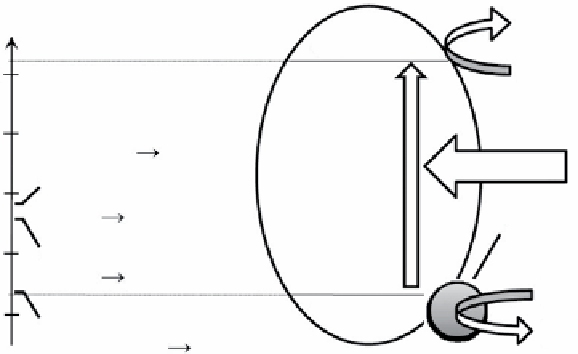