Environmental Engineering Reference
In-Depth Information
e
-
/H
+
O
2
CB
e
-
e
-
-0.5V
O
2
-
e
-
e
-
H
2
O
2
hν
3
hν
1
hν
2
OH
•
h
+
h
+
OH
-
+2.7
h
+
VB
h
+
OH
•
figure 2.1
TiO
2
photocatalyst's band-gap modification for undoped (hν
1
), metal doping (hν
2
), and nonmetal doping (hν
3
).
several years ago, sato discovered that the addition of NH
4
OH in a titania sol-gel formulation followed by calcination of the
precipitated powder at temperatures of 300 °C and higher resulted in a material that exhibited visible light sensitization [38]
.
It was not until a few years ago that a first explanation of this phenomenon was provided by Asahi and coworkers who reported
that nitrogen-doped TiO
2
could absorb in the visible light. It was a yellowish material prepared by sputtering a TiO
2
target under
a N
2
(40%)/Ar gas mixture followed by annealing under N
2
at 550 °C for 4 h, and it was photocatalytically active for the degra-
dation of methylene blue and acetaldehyde under visible light [39-42]
.
Nitrogen is one of the most widely reported dopants for shifting TiO
2
spectral absorption into the visible range. substitution
of N in the titania lattice has been proposed to contribute to a narrow band gap by merging nitrogen and oxygen 2p states on the
top of the valence band as nitride (Ti-N) or oxynitride (Ti-O-N). Another theory is the formation of oxyanion species at
interstitial lattice sites, where N is bonded to one or more oxygen atoms creating localized intergap states. Both arrangements
are considered to change the energy photothreshold responsible for the red shift of the optical absorption toward the visible
spectra region [43].
several recent studies in this emerging field of N-doped TiO
2
catalyst activated by visible light deal with the fundamental
physics to explain the electronic properties of N-doped TiO
2
[44-46] and provide solid evidence for the promising properties
of this environmentally “green” material [47, 48]. However, some other reports have suggested that anion-doped TiO
2
photo-
catalysts have low efficiency due to charge carrier recombination from anion doping [49] and that metal ion modification influ-
ences light absorption and photoreactivity of TiO
2
acting as electron/hole traps [50].
silver-, vanadium-, iron-, and palladium-modified nitrogen-doped TiO
2
have been tested to enhance titania's PC performance
in inactivating Gram-negative bacteria, such as
Escherichia coli
,
Pseudomonas aeruginosa
, and
Prevotella intermedia
[50-55],
as well as some Gram-positive bacteria, such as Methicillin-resistant
Staphylococcus aureus
(MRsA),
Staphylococcus epider-
midis
,
Staphylococcus saprophyticus
,
Streptococcus pyogenes
, and
Saccharomyces cerevisiae
[56-59], and a few protozoa,
such as
Tetraselmis suecica
,
Amphidinium carterae
,
Chlorella vulgaris
[60, 61], in water
.
Nonmetallic ion doping has also been shown to be effective to induce modifications of the electronic structure of TiO
2
by
creating surface oxygen vacancies due to charge compensation between the nonmetallic ion and Ti
4+
but without producing a
significant change in the optical absorption of the photocatalyst [37, 62]
.
Moreover, codoping of TiO
2
with nitrogen and fluorine
has demonstrated high PC activity in the visible region with beneficial effects induced by both dopants [63-65]. Huang et al.
confirmed strong visible light absorption and high PC activity of N-fTiO
2
for
p
-chlorophenol and rhodamine B degradation
under visible light irradiation [64]. xie et al. effectively decomposed methyl orange with a visible light-activated N-f-TiO
2
photocatalyst [65]. Both attributed their findings to the synergistic effect of nitrogen and fluorine doping. More recently,
Dionysiou's group has shown the effectiveness of N-TiO
2
in removing cyanobacterial toxins in water by using visible radiation
[37, 66]
.
Castillo-Ledezma et al. have recently demonstrated the capability of N-f-TiO
2
in inactivating
E. coli
using solar radi-
ation under several different reaction conditions, including pH value and radiation wavelengths (visible and UV + visible), and
they showed that the N-f-TiO
2
process possesses higher efficiency than the regular TiO
2
and solar disinfection processes [2].
similar results were found by Wong et al. for nitrogen-doped TiO
2
in inactivating
E. coli
and several other pathogenic microor-
ganisms [67]. They also found that proteins and light-absorbing contaminants reduce the bacterial activity of the photocatalyst
as a result of their light-shielding effects. Liu et al. found that extracellular polymeric substances (EPs) generated by some
heterotrophic bacteria play an important role in controlling the kinetics of the solar-induced PC process by generating a
protective layer against the presence of photogenerated reactive oxygen species (ROs) during the photocatalyst activation [68].
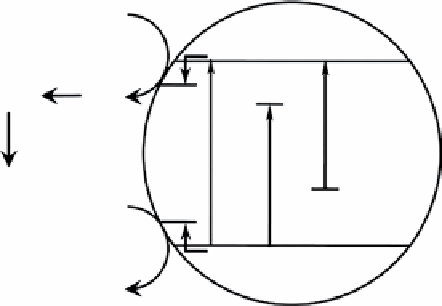