Environmental Engineering Reference
In-Depth Information
224
420
040
441
11-1
011
022
021
(a)
(c)
(e)
120
121
220
012
50 nm
50 nm
100 nm
(b)
(d)
(f)
25
25
25
20
20
20
15
15
15
10
10
10
5
5
5
0
5
0
0
678910
Particle size (nm)
11 12 13 14 15
6
78910
11
12
13
3
45678910
11
Particle size (nm)
Particle size (nm)
fiGUre 20.5
TeM images of silica (a), titania (c), and zirconia (e) nanoparticles biosynthesized using the fungus
F. oxysporum.
Insets in
(a), (c), and (e) correspond to the selected area electron diffraction patterns recorded from the particles shown in the respective main figure.
The particle size histograms of the silica, titania, and zirconia particles presented in images (a), (c), and (e) are shown in (b), (d), and (f),
respectively. Images reprinted from Refs. [107, 108]. Reproduced by permission of The Royal Society of chemistry (RSc).
(SiF
6
2-
, TiF
6
2-
, and ZrF
6
2-
ions) employed in these studies [107, 108]. However, the evolutionary role of these hydrolytic
enzymes during the life cycle of the fungus is still unclear. Although the morphology of these oxides are similar, magnetite
nanoparticles synthesized by
F. oxysporum
and
Verticillium
sp. were significantly different as the former fungus synthe-
sized particles of 20-40 nm in diameter, while the latter yielded a super-assembly of smaller magnetite nanoparticles into
cubical structures, with an edge length of 100-400 nm [109]. In addition to fungi, several bacterial species, plants, and
actinomycete have also been reported for their ability to synthesize magnetite nanoparticles [111-114] that are outlined in
Table 20.2. Recent studies have shown the ability of
F. oxysporum
for the synthesis of extremely small (5-8 nm) bismuth
oxide nanoparticles at room temperature [115]. This material typically showed a mixture of monoclinic and tetragonal
phase, which is quite remarkable given that these materials exhibit a tetragonal phase when heated above 700°c. Incidentally,
several plant species have also been known to accumulate and synthesize simple (Fe
3
O
4
and ZnO) [116, 117] and complex
(laMnO
3
) [118] metal oxide nanomaterials, which are either in response to high metal stress environment or synthesized
by deliberately exposing them to metal ions.
In addition to the ability of fungal species to synthesize simple metal oxides, some species of fungi were also able to synthe-
size complex metal oxides such as barium titanate and chemically “difficult-to-synthesize” multifunctional cuAlO
2
nanopar-
ticles [119]. The ability of fungus
F. oxysporum
to synthesize ultra-small sub-10 nm tetragonal barium titanate nanoparticles in
the presence of barium acetate and potassium hexafluorotitanate precursors is quite fascinating as this fungus is not known to
encounter these metal ions in their natural habitat [110]. It is important to highlight that BaTiO
3
is a technologically important
material, however this material loses its ferroelectric properties in its nano-form, as the BaTiO
3
crystals transform from
tetragonal (bulk) to cubic (nano) phase [120]. However, the ultra-small BaTiO
3
nanocrystals formed by fungus
F. oxysporum
could retain their ferroelectric properties even below sub-10 nm size regime, as was demonstrated through room-temperature
ferroelectricity and ferroelectric-relaxor behavior studies. Additionally, Kelvin probe microscopy further enabled the electrical
writing and thereafter reading the information on individual BaTiO
3
nanoparticles synthesized by this fungus. This opens up
exciting opportunities in terms of synthesizing organic-inorganic hybrid biogenic materials on a large scale for applications in
the field of electronics.
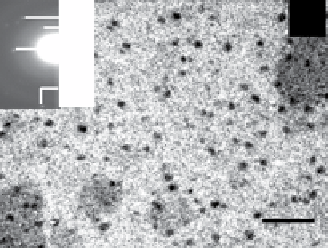
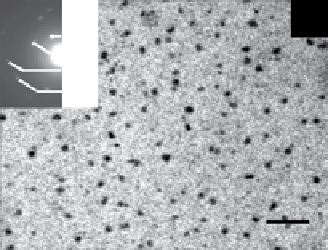
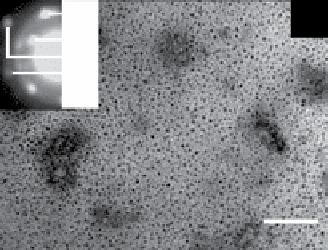


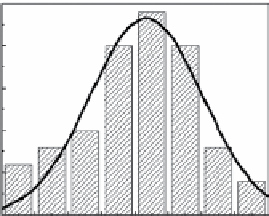